
Quantum physics: what is really real?
- Transfer
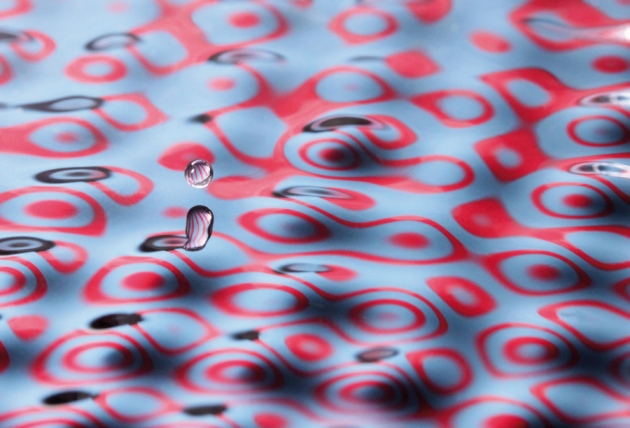
According to Owen Maruni, a physicist at Oxford University, since the advent of quantum theory in the 1900s, everyone has been talking about the strangeness of this theory. How does it allow particles and atoms to move in several directions simultaneously, or simultaneously rotate clockwise and counterclockwise. But you can’t prove anything with words. “If we tell the public that quantum theory is very strange, we need to verify this statement experimentally,” says Maruni. “Otherwise, we are not doing science, but are talking about all kinds of squiggles on the board.”
This is what led Marouni’s associates to develop a new series of experiments to uncover the essence of the wave function — the mysterious essence that underlies quantum oddities. On paper, the wave function is simply a mathematical object, denoted by the letter psi (Ψ) (one of those same squiggles), and is used to describe the quantum behavior of particles. Depending on the experiment, the wave function allows scientists to calculate the probability of observing an electron in a particular place, or the chances that its spin is oriented up or down. But mathematics does not say what a wave function really is. Is this something physical? Or just a computing tool that allows you to work with observer ignorance about the real world?
The tests used to answer the question are very subtle, and they still have to give a definite answer. But researchers are optimistic that the denouement is close. And finally, they will be able to answer questions that have plagued all decades. Can a particle really be in many places at the same time? Is the Universe constantly divided into parallel worlds, in each of which our alternative version exists? Is there anything at all called objective reality?
“Such questions sooner or later come to anyone,” says Alessandro Fedricci, a physicist at the University of Queensland (Australia). "What is really real?"
Disputes about the essence of reality began even when physicists found out that a wave and a particle are only two sides of the same coin. A classic example is an experiment with two slots, where individual electrons shoot at a barrier with two slots: the electron behaves as if it passes through two slits at the same time, creating a striped pattern of interference from its other side. In 1926, the Austrian physicist Erwin Schrödinger invented a wave function to describe this behavior and derived an equation that made it possible to calculate it for any situation. But neither he nor anyone else could tell anything about the nature of this function.
Grace in ignorance
From a practical point of view, its nature is not important. The Copenhagen interpretation of quantum theory, created in the 1920s by Niels Bohr and Werner Heisenberg, uses the wave function simply as a tool to predict the results of observations, allowing you not to think about what happens in reality. “You can’t blame physicists for such a behavior model, shut up and count, because it has led to significant breakthroughs in nuclear and atomic physics, solid state physics and elementary particle physics,” says Gene Brickmont, a specialist in statistical physics at Catholic University of Belgium. “Therefore, people are advised not to worry about fundamental issues.”
But some are still worried. By the 1930s, Einstein rejected the Copenhagen interpretation, not least because it allowed two particles to confuse their wave functions, which led to a situation in which the measurements of one of them could instantly give the state of the other, even if they were separated by huge distances. In order not to put up with this “frightening interaction at a distance”, Einstein preferred to believe that the wave functions of the particles were incomplete. He said that it is possible that particles have some hidden variables that determine the measurement result, which were not noticed by quantum theory.
Experiments have since demonstrated the performance of frightening interactions at a distance, which rejects the concept of hidden variables. but this did not stop the other physicists from interpreting them in their own way. These interpretations are divided into two camps. Some agree with Einstein that the wave function reflects our ignorance. This is what philosophers call psi-epistemic models. And others see the wave function as the real thing - psi-ontic models.
To understand the difference, imagine the Schroдингdinger mental experiment described in 1935 in a letter to Einstein. The cat is in a steel box. The box contains a sample of radioactive material that has a 50% chance of emitting a decay product in one hour, and an apparatus that poisons a cat if this product is detected. Since radioactive decay is a quantum-level event, Schrödinger writes, the rules of quantum theory say that at the end of the hour, the wave function of the box's insides should be a mixture of a dead and a living cat.
“Roughly speaking,” Fedricci gently puts it, “in the psi-epistemic model, the cat in the box is either alive or dead, and we just don’t know this because the box is closed.” And in most psi-ontic models there is agreement with the Copenhagen interpretation: until the observer opens the box, the cat will be both alive and dead.
But here the argument comes to a standstill. Which of the interpretations is true? It is difficult to answer this question experimentally, since the difference between the models is very subtle. In essence, they must predict the same quantum phenomenon as the very successful Copenhagen interpretation. Andrew White, a physicist at the University of Queensland, said that during his 20-year career in quantum technology, "this task was like a huge smooth mountain without ledges, which could not be approached."
Everything changed in 2011, with the publication of the theorem on quantum measurements, which seems to have eliminated the “wave function as ignorance” approach. But upon closer examination, it turned out that this theorem leaves enough space for their maneuver. However, it inspired physicists to seriously think about ways to resolve the dispute by testing the reality of the wave function. Maruni has already developed an experiment, which in principle is workable, and he and his colleagues soon found a way to make it work in practice. The experiment was conducted last year by Fedricci, White and others.
To understand the idea of the test, imagine two decks of cards. In one there are only reds, in the other - only aces. “They give you a card and ask you to determine which deck it comes from,” says Martin Ringbauer, a physicist at the same university. If this is a red ace, “an intersection happens, and you cannot say it for sure.” But if you know how many cards in each deck, you can calculate how often this ambiguous situation will occur.
Physics are in danger
The same ambiguity happens in quantum systems. It is not always possible to find out with one measurement, for example, how a photon is polarized. “In real life, it's easy to distinguish the west from the direction just south of the west, but in quantum systems it's not that simple,” says White. According to the standard Copenhagen interpretation, it makes no sense to ask about polarization, because the question has no answer - until another measurement determines the answer exactly. But according to the “wave function as ignorance” model, the question makes sense - it’s just that the experiment, as well as the one with the decks of cards, lacks information. As with cards, it is possible to predict how many ambiguous situations can be explained by such ignorance, and compare with the large number of ambiguous situations allowed by standard theory.
This is exactly what Fedricci checked with the team. The group measured polarization and other properties in the beam of photons, and found the level of intersection, which cannot be explained by models of "ignorance." The result supports an alternative theory - if objective reality exists, then there is a wave function. “It’s impressive that the team was able to solve such a difficult task with such a simple experiment,” says Andrea Alberti, a physicist at the University of Bonn (Germany).
The conclusion has not yet been carved in granite: since the detectors captured only a fifth of the photons used in the test, we have to assume that the lost photons behaved in exactly the same way. This is a strong assumption, and now the group is working to reduce losses and produce a more specific result. At this time, the Maruni team at Oxford is working with the University of New South Wales (Australia) to repeat this experiment with ions that are easier to track. “In the next six months, we will have an undeniable version of this experiment,” says Maruni.
But even if success awaits them and the “wave function as reality” models win, then these models have different options. Experimenters will have to choose one of them.
One of the earliest interpretations was made in the 1920s by the Frenchman Louis de Broglie, and expanded in the 1950s by the American David Bohm. According to the Broglie-Bohm models, particles have a specific location and properties, but they are driven by a certain “pilot wave”, which is defined as a wave function. This explains the experiment with two slits, since the pilot wave can pass through both slots and give an interference picture, although the electron itself, attracted by it, passes through only one of the two slits.
In 2005, this model received unexpected support. Physicists Emmanuel Fort, now working at the Langevin Institute in Paris, and Yves Kodier from the University of Paris Didro asked students a simple task, in their opinion: to conduct an experiment in which drops of oil falling on a tray will merge due to vibrations of the tray. To the surprise of everyone around the drops, waves began to form when the tray vibrated at a certain frequency. “The drops began to move independently along their own waves,” says Fort. "It was a dual object - a particle drawn by a wave."
Since then, Fort and Code have shown that such waves can conduct their particles in an experiment with two slits exactly as the pilot wave theory predicts, and can reproduce other quantum effects. But this does not prove the existence of pilot waves in the quantum world. “We were told that such effects are impossible in classical physics,” says Fort. “And then we showed that they are possible.”
Another set of models based on reality, developed in the 1980s, is trying to explain the strong difference in properties between large and small objects. “Why electrons and atoms can be in two places at the same time, but tables, chairs, people and cats cannot,” says Angelo Basi, physicist at the University of Trieste (Italy). Known as “collapse models,” these theories say that the wave functions of individual particles are real, but can lose their quantum properties and bring the particle into a certain position in space. The models are constructed so that the chances of such a collapse are extremely small for an individual particle, so that quantum effects dominate at the atomic level. But the probability of collapse increases rapidly when particles are combined, and macroscopic objects completely lose their quantum properties and behave according to the laws of classical physics.
One way to check this is to look for quantum effects in large objects. If the standard quantum theory is true, then there are no size restrictions. And physicists have already conducted an experiment with two slits using large molecules. But if the collapse models are true, then quantum effects will not be visible if a certain mass is exceeded. Different groups plan to search for this mass using cold atoms, molecules, metal clusters and nanoparticles. They hope to discover results in the next ten years. “What's cool with these experiments is that we will put the quantum theory to exact tests where it has not been tested yet,” says Maruni.
Parallel Worlds
One model “wave function as reality” is already known and loved by science fiction writers. This is a world-wide interpretation developed in the 1950s by Hugh Everett, who was then a student at Princeton University in New Jersey. In this model, the wave function determines the development of reality so strongly that, with each quantum measurement, the Universe splits into parallel worlds. In other words, opening a box with a cat, we give birth to two Universes - one with a dead cat, and the other with a living one.
It is difficult to separate this interpretation from the standard quantum theory, since their predictions coincide. But last year, Howard Wiseman of the University of Griffith in Brisbane and colleagues proposed a model of the multivers that can be tested. In their model there is no wave function - particles obey classical physics, Newton's laws. And the strange effects of the quantum world appear because there are repulsive forces between particles and their clones in parallel universes. “The repulsive force between them generates waves propagating across all parallel worlds,” says Wiseman.
Using a computer simulation in which 41 universes interacted, they showed that the model roughly reproduces several quantum effects, including particle trajectories in an experiment with two slits. With an increase in the number of worlds, the pattern of interference tends to the real. Since the predictions of the theory vary depending on the number of worlds, says Wiseman, you can check whether the multiverse model is right - that is, that there is no wave function, and reality works according to classical laws.
Since the wave function is not needed in this model, it will remain viable even if future experiments exclude models with “ignorance”. In addition to it, other models will survive, for example, the Copenhagen interpretation, which claim that there is no objective reality, but only calculations.
But then, as White says, this question will be the object of study. And although no one knows how to do it yet, “what would be really interesting is to develop a test that checks whether we have any objective reality”.