
The story of one discovery
In the second year of the institute, when we were told the “History of Science”, I remember listening to a student who forgot to turn off the crucible and made a discovery, or one famous scientist who got an apple on his head, and thought it was a fairy tale, and in modern this does not happen to science. In principle, if you look at the publications in top physical journals, you can see that all of them are the result of long hard digging in one direction. Novoselov and Game did not even receive the Nobel Prize for the discovery, as such, but for the "systematic study of properties." But, nevertheless, discoveries in modern science still happen, and I want to talk about one of them, being its co-author.
From the beginning - a short excursion into the field of physics and nanotechnology, the core of which I deal with is micro- and nanotribology. Tribology itself is a science of a very respectable age, which deals with friction and wear. It would seem that everything has been known for a long time in this area - he poured more grease, and no friction. And to study here, from a scientific point of view, there is nothing special. But with the development of microminiaturization, tribology got a triple breath. Because the methods of the macrocosm (pouring a bucket of oil) at the micro level no longer work - and not because everything will just drown, can you add oil and drop by drop?
The problem is that as the size of the moving parts decreases, the surface contribution increases. And all sorts of surface effects, which are insignificant at the macro level, begin to dominate at the micro level. In particular, surface tension. Therefore, when components are reduced, after a certain limit, grease cannot be used. And dry friction appears on the scene. For example, the coefficient of dry friction of silicon over silicon (the most common material for MEMS) reaches 0.7. Those. 70% of the power of such an engine will go simply to turn the rotor. We need to somehow deal with this. The obvious way is to apply some solidlow friction coating. Since we are talking about micro-components, and the thickness of the coating should be very small - usually we are talking about tens of nanometers, but there are also ultrathin coatings with a thickness of 1–2 nm. In principle, there is a fair amount of coatings that can be used to reduce friction and wear — soft metals, self-orientating organic molecules, graphene, and diamond-like films. There are a lot of materials, but they all have one or another shortcoming, and they have not yet come up with any universal one.
From this list, perhaps, diamond-like coatings (DLC) are most known. Moreover, they can be equally successfully applied both at the micro and macro levels. So, Hyundai currently uses DLC to cover the surface of valves in engines installed on top models of cars. It is planned to use DLC in HDD for hardening the seating surfaces of hydrodynamic bearings. You can find hundreds of other real-life DLC applications, including coating on the cutting edge of shaving blades. In most cases, magnetron sputtering is used to apply the DLC — a well-known and established method. But, as always, there are nuances. The most important thing - all this is very expensive. There are also purely technical problems, such as a high level of internal stresses, sensitivity to humidity, etc.
Together with colleagues from my alma mater, we have been developing one, we can say an alternative technology for several years - the application of diamond-like films by an ion beam, in which not 60 atomic carbon is used as the material, but C 60 fullerene molecules . Fullerene is ionized, accelerates to 5 keV and peels on the substrate. In this case, the molecules break up, and an amorphous structure with interesting properties is formed from the debris. Details can be found in this article.. This method has its advantages, in particular, our films are not afraid of moisture, well, the use of an ion beam allows coating on objects of arbitrary shape, which is somewhat difficult in the case of magnetron sputtering. The disadvantage of our films is a rather high level of their own stresses. The film seeks to expand, to occupy a larger volume than it has. This leads to unpleasant consequences - if you apply such a film to a thin substrate, the substrate may bend. If the substrate is thicker, and the adhesion between the film and the substrate is not good enough - the film simply peels off.
We had the idea to dilute the solid mass of the DLC with something soft to compensate for internal stresses. And, since fullerene was used as the main material, it was added. It turned out that if, parallel to the ion beam, a molecular beam is directed onto the substrate, the result is a kind of nano-composite in which the fullerene molecules are surrounded by solid amorphous carbon. As expected, the stress level in such a film was significantly less. Generally speaking, we did not find any stresses. Of course, the film hardness also decreased - if the film deposited from the ion beam is characterized by values of 50–60 GPa, then the nano-composite showed 25–30 GPa. But this is still quite a lot - for example, the hardness of single-crystal silicon is ~ 10 GPa. Hooray, the problem is solved. Here, in the process of measuring hardness,
But, before we get to the point, you need to make another digression. Talk about how the hardness of films is measured. In principle, the method is the same - we take a calibrated diamond pyramid and press it into the surface with a certain effort. The softer the material, the deeper the pyramid will be pressed. We measure the size of the print - we get hardness. All this is easy when you need to measure the hardness of the rail. And it becomes difficult when it comes to films with a thickness of 100 nm. For these purposes, a nanoindentation method was developed.(nanoindentation, depth sensing indentation). The bottom line is that we gradually increase the load on the pyramid (indenter) and at the same time fix the penetration depth. The linear law of loading and unloading is usually used. Well, a special pyramid is needed. In our case, this is a trihedral pyramid with a tip diameter of 100 nm.
As a result of a “controlled piercing,” for example, of a soft fullerene film, we get this curve:
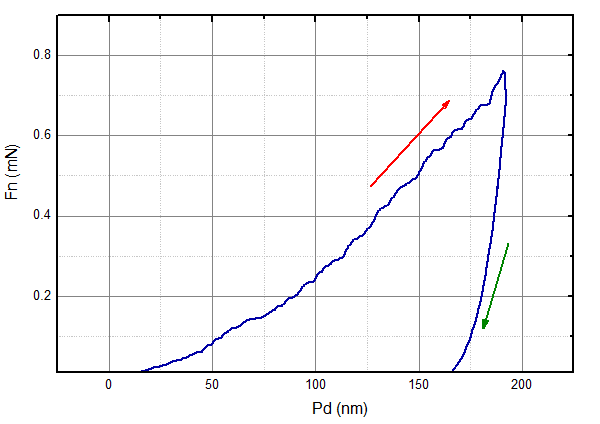
Here, the X axis is the penetration depth of the indenter (in nanometers), Y is the force applied to the indenter. The red arrow indicates the direction of the load, green - unloading. Depth of penetration depends on hardness. The softer the material, the deeper the indenter penetrates at the same load. In this case, the elasticity (Young's modulus) can be calculated from the angle of inclination of the load curve. The load and unload curves do not coincide as a result of plastic deformation at the contact point. If we examine the fingerprint using an atomic force microscope, we get this picture:
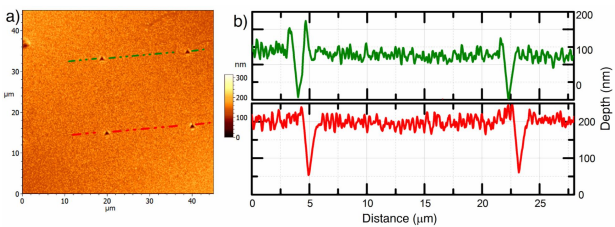
Left - top view, right - sections along the red and green lines. It is clearly seen that the indenter is a trihedral pyramid :). In the case of elastic materials, such as rubber, the load curve will coincide with the unloading curve, because in this case only elastic deformation takes place, to a certain limit, of course, well, there will be no imprint on the surface. The case when the unloading curve will lie higher than the load curve, in principle, is not possible.
Well, once, such an “impossible” curve was recorded experimentally (Figure d):

At first, I just decided that it was some kind of glitch in the device. Then I checked again. Reproduced. Did not believe. Then he began to understand. As it turned out, this phenomenon is characteristic of nano-composites consisting of a mixture of fullerene molecules and solid amorphous carbon. Depending on the speed at which the load and unload during the test, the curve changes its shape. When we press quickly, we get a typical picture for a hard film (a). We press slowly - we get "that which cannot be." Obviously, at a low burst speed, some additional driving force arises in the film, which pushes the indenter back. But which one?
A detailed analysis showed that in the case of “anomalous” indentation, instead of a print, a hill several tens of nanometers high (a, b) is formed:
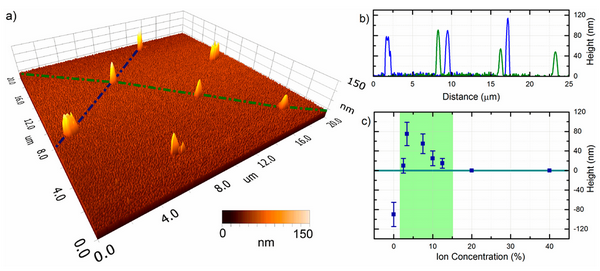
A detailed analysis showed that the height of the "hills" depends on the ratio of ionic and molecular beams in the manufacturing process (s).
Obviously, under load, the material swells, which leads to the expulsion of the indenter and the formation of hills instead of prints. So due to what? With the combined use of ion and molecular beams, the polymerization of fullerene molecules occurs. In the normal state, they are connected by weak Van der Waals bonds. However, if they are thoroughly “kicked”, a much stronger covalent bond is formed between two neighboring molecules. These two types of bonds, in addition to strength, differ in length. The covalent bond is shorter and the polymerized molecules are packed denser. When pierced, polymer complexes at the point of contact are deformed, and covalent bonds are destroyed. As a result, tightly packed molecules tend to move away from each other, which leads to an increase in volume, filling of the print and the formation of a hill. Why is this effect observed only with slow indentation? We believe that the exit of depolymerized molecules to the surface is a diffusion process, and with rapid indentation they simply do not have enough time.
In addition to “self-healing” surfaces, such nano-composite films exhibit another interesting property - dynamic hardness. The film is very hard in the event of an impact load, while being relatively soft and pliable in the case of a load that is constant or slowly increasing. Why this is needed - we have not yet figured out, while in the air thoughts like "nano-body armor for nano-robots" are worn. Any ideas?
A more detailed description can be found in this article . She's on the sci-hub: http://pubs.acs.org.sci-hub.org/doi/abs/10.1021/nl500321g .
______________________
From the beginning - a short excursion into the field of physics and nanotechnology, the core of which I deal with is micro- and nanotribology. Tribology itself is a science of a very respectable age, which deals with friction and wear. It would seem that everything has been known for a long time in this area - he poured more grease, and no friction. And to study here, from a scientific point of view, there is nothing special. But with the development of microminiaturization, tribology got a triple breath. Because the methods of the macrocosm (pouring a bucket of oil) at the micro level no longer work - and not because everything will just drown, can you add oil and drop by drop?
The problem is that as the size of the moving parts decreases, the surface contribution increases. And all sorts of surface effects, which are insignificant at the macro level, begin to dominate at the micro level. In particular, surface tension. Therefore, when components are reduced, after a certain limit, grease cannot be used. And dry friction appears on the scene. For example, the coefficient of dry friction of silicon over silicon (the most common material for MEMS) reaches 0.7. Those. 70% of the power of such an engine will go simply to turn the rotor. We need to somehow deal with this. The obvious way is to apply some solidlow friction coating. Since we are talking about micro-components, and the thickness of the coating should be very small - usually we are talking about tens of nanometers, but there are also ultrathin coatings with a thickness of 1–2 nm. In principle, there is a fair amount of coatings that can be used to reduce friction and wear — soft metals, self-orientating organic molecules, graphene, and diamond-like films. There are a lot of materials, but they all have one or another shortcoming, and they have not yet come up with any universal one.
From this list, perhaps, diamond-like coatings (DLC) are most known. Moreover, they can be equally successfully applied both at the micro and macro levels. So, Hyundai currently uses DLC to cover the surface of valves in engines installed on top models of cars. It is planned to use DLC in HDD for hardening the seating surfaces of hydrodynamic bearings. You can find hundreds of other real-life DLC applications, including coating on the cutting edge of shaving blades. In most cases, magnetron sputtering is used to apply the DLC — a well-known and established method. But, as always, there are nuances. The most important thing - all this is very expensive. There are also purely technical problems, such as a high level of internal stresses, sensitivity to humidity, etc.
Together with colleagues from my alma mater, we have been developing one, we can say an alternative technology for several years - the application of diamond-like films by an ion beam, in which not 60 atomic carbon is used as the material, but C 60 fullerene molecules . Fullerene is ionized, accelerates to 5 keV and peels on the substrate. In this case, the molecules break up, and an amorphous structure with interesting properties is formed from the debris. Details can be found in this article.. This method has its advantages, in particular, our films are not afraid of moisture, well, the use of an ion beam allows coating on objects of arbitrary shape, which is somewhat difficult in the case of magnetron sputtering. The disadvantage of our films is a rather high level of their own stresses. The film seeks to expand, to occupy a larger volume than it has. This leads to unpleasant consequences - if you apply such a film to a thin substrate, the substrate may bend. If the substrate is thicker, and the adhesion between the film and the substrate is not good enough - the film simply peels off.
We had the idea to dilute the solid mass of the DLC with something soft to compensate for internal stresses. And, since fullerene was used as the main material, it was added. It turned out that if, parallel to the ion beam, a molecular beam is directed onto the substrate, the result is a kind of nano-composite in which the fullerene molecules are surrounded by solid amorphous carbon. As expected, the stress level in such a film was significantly less. Generally speaking, we did not find any stresses. Of course, the film hardness also decreased - if the film deposited from the ion beam is characterized by values of 50–60 GPa, then the nano-composite showed 25–30 GPa. But this is still quite a lot - for example, the hardness of single-crystal silicon is ~ 10 GPa. Hooray, the problem is solved. Here, in the process of measuring hardness,
But, before we get to the point, you need to make another digression. Talk about how the hardness of films is measured. In principle, the method is the same - we take a calibrated diamond pyramid and press it into the surface with a certain effort. The softer the material, the deeper the pyramid will be pressed. We measure the size of the print - we get hardness. All this is easy when you need to measure the hardness of the rail. And it becomes difficult when it comes to films with a thickness of 100 nm. For these purposes, a nanoindentation method was developed.(nanoindentation, depth sensing indentation). The bottom line is that we gradually increase the load on the pyramid (indenter) and at the same time fix the penetration depth. The linear law of loading and unloading is usually used. Well, a special pyramid is needed. In our case, this is a trihedral pyramid with a tip diameter of 100 nm.
As a result of a “controlled piercing,” for example, of a soft fullerene film, we get this curve:
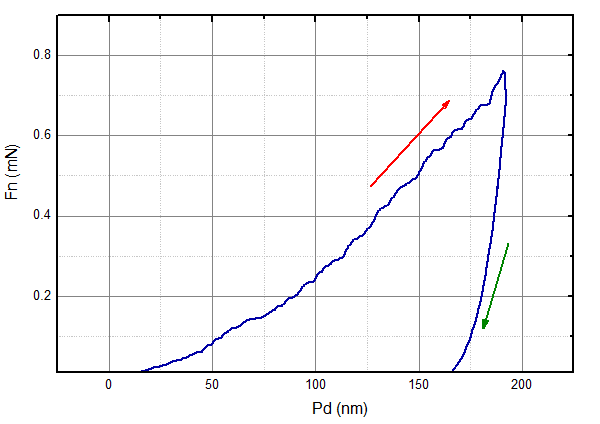
Here, the X axis is the penetration depth of the indenter (in nanometers), Y is the force applied to the indenter. The red arrow indicates the direction of the load, green - unloading. Depth of penetration depends on hardness. The softer the material, the deeper the indenter penetrates at the same load. In this case, the elasticity (Young's modulus) can be calculated from the angle of inclination of the load curve. The load and unload curves do not coincide as a result of plastic deformation at the contact point. If we examine the fingerprint using an atomic force microscope, we get this picture:
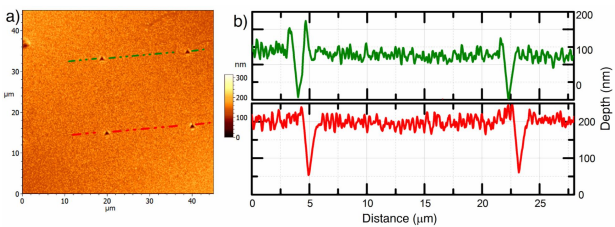
Left - top view, right - sections along the red and green lines. It is clearly seen that the indenter is a trihedral pyramid :). In the case of elastic materials, such as rubber, the load curve will coincide with the unloading curve, because in this case only elastic deformation takes place, to a certain limit, of course, well, there will be no imprint on the surface. The case when the unloading curve will lie higher than the load curve, in principle, is not possible.
Well, once, such an “impossible” curve was recorded experimentally (Figure d):

At first, I just decided that it was some kind of glitch in the device. Then I checked again. Reproduced. Did not believe. Then he began to understand. As it turned out, this phenomenon is characteristic of nano-composites consisting of a mixture of fullerene molecules and solid amorphous carbon. Depending on the speed at which the load and unload during the test, the curve changes its shape. When we press quickly, we get a typical picture for a hard film (a). We press slowly - we get "that which cannot be." Obviously, at a low burst speed, some additional driving force arises in the film, which pushes the indenter back. But which one?
A detailed analysis showed that in the case of “anomalous” indentation, instead of a print, a hill several tens of nanometers high (a, b) is formed:
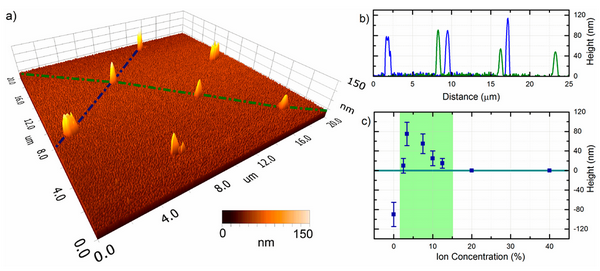
A detailed analysis showed that the height of the "hills" depends on the ratio of ionic and molecular beams in the manufacturing process (s).
Obviously, under load, the material swells, which leads to the expulsion of the indenter and the formation of hills instead of prints. So due to what? With the combined use of ion and molecular beams, the polymerization of fullerene molecules occurs. In the normal state, they are connected by weak Van der Waals bonds. However, if they are thoroughly “kicked”, a much stronger covalent bond is formed between two neighboring molecules. These two types of bonds, in addition to strength, differ in length. The covalent bond is shorter and the polymerized molecules are packed denser. When pierced, polymer complexes at the point of contact are deformed, and covalent bonds are destroyed. As a result, tightly packed molecules tend to move away from each other, which leads to an increase in volume, filling of the print and the formation of a hill. Why is this effect observed only with slow indentation? We believe that the exit of depolymerized molecules to the surface is a diffusion process, and with rapid indentation they simply do not have enough time.
In addition to “self-healing” surfaces, such nano-composite films exhibit another interesting property - dynamic hardness. The film is very hard in the event of an impact load, while being relatively soft and pliable in the case of a load that is constant or slowly increasing. Why this is needed - we have not yet figured out, while in the air thoughts like "nano-body armor for nano-robots" are worn. Any ideas?
A more detailed description can be found in this article . She's on the sci-hub: http://pubs.acs.org.sci-hub.org/doi/abs/10.1021/nl500321g .
______________________