Ultra high energy neutrinos emitted by radiant galaxies at the other end of the Universe
- Transfer

An artistic depiction of how a blazar accelerates protons that produce pions, which in turn generate neutrinos and gamma rays. Neutrinos are always the result of hadron reactions. Gamma rays can appear in both hadronic and electromagnetic interactions.
One of the greatest mysteries of science is the definition of not only objects existing in the Universe, but also the sources of signals that we fix here on Earth. For more than a hundred years we have known that cosmic rays have been plying the Universe: high-energy particles, whose sources are far beyond the limits of our Galaxy. And although some of the sources of these particles are already identified, most of them, including those that are the most energetic, remained a mystery.
And so, this situation has changed. On September 22, 2017, the IceCube collaboration detected ultrahigh-energy neutrinos arriving at the South Pole and was able to determine their source. When several telescopes operating in the gamma range were directed to the same point, they not only saw the signal, but also recognized the blazar flashing right at that moment . Finally, humanity has discovered at least one source creating these ultra-energy cosmic particles.
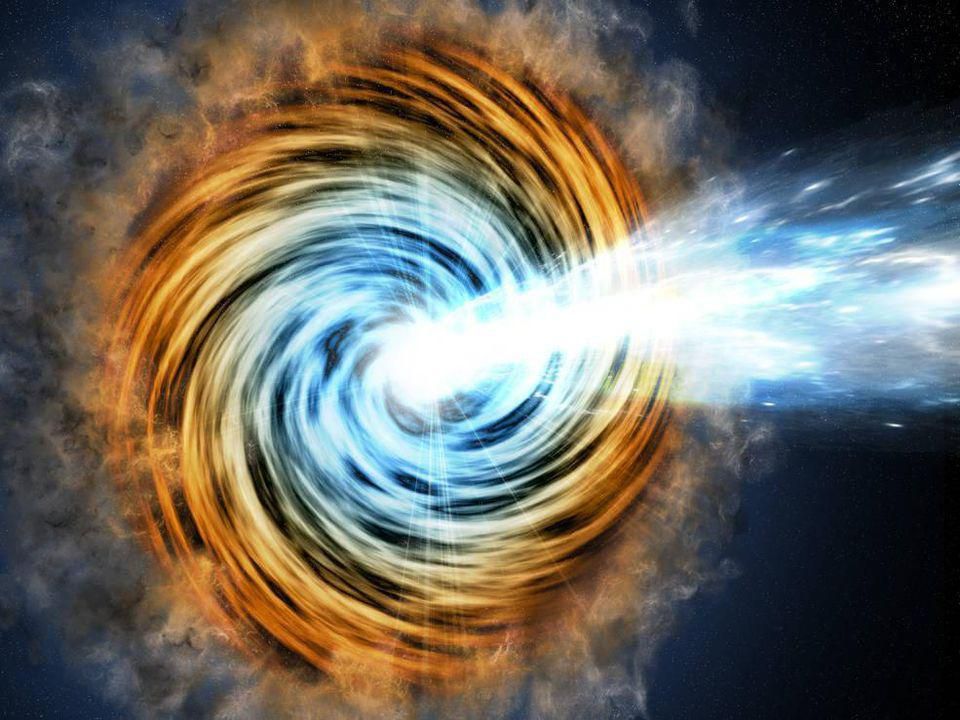
When black holes devour matter, they create an accretion disk and jets flying from both poles, which are perpendicular to it. When the jet of a supermassive black hole points to us, we call it the blazar, and in a particular case it was the BL object of the Lizard. Now they are considered the main source of cosmic rays and high-energy neutrinos. The
Universe, wherever we look, is filled with things that can be observed and with which you can interact. Matter clumps, forming galaxies, stars, planets and even people. Radiation flows through the Universe, covering the entire electromagnetic spectrum. And in every cubic centimeter of space you can find hundreds of ghostly particles with a tiny mass, known as neutrinos.
At the very least, they could be found if they often interact with normal matter, with which we know how to manage. But instead, neutrinos can pass through a wall of lead thickness in a light year, and have a 50/50 chance of colliding with any particle of it. Several decades after their existence was assumed in 1930, we could not detect them.

Experimental nuclear reactor RA-6 , demonstrating the characteristic Cherenkov radiation caused by particles moving faster than light in water. Neutrinos (or, more precisely, antineutrinos), the hypothesis of the existence of which Pauli first put forward in 1930, were discovered in a similar nuclear reactor in 1956.
In 1956, we first discovered them by placing the detectors near nuclear reactors, a few meters from the place of their appearance. In the 1960s, we built large enough detectors — underground, protected from other contaminating particles — to find neutrinos appearing in the Sun, and those generated by the collision of cosmic rays with the atmosphere.
Then in 1987 only a pleasant surprise in the form of a supernova explosion near us allowed us to detect the neutrinos flying from there. Experiments that worked with a completely different purpose , found neutrinos from SN 1987A , and opened the era of astronomy of several "messengers". As far as we could tell, neutrinos flew through the Universe with energies indistinguishable from the speed of light.

1987n supernova remnants located in the Large Magellanic Cloud, 165,000 light-years from us. The fact that neutrinos arrived a few hours before the first signal told us more about the time it took the light to propagate through the star's layers than about the speed of movement of the neutrinos, which was indistinguishable from the speed of light. Apparently, neutrinos, light and gravity move with the same speed.
For about 30 years, the neutrinos from this supernova have remained the only confirmed neutrinos that have come from outside the solar system, not to mention the limits of the galaxy. But this does not mean that we did not take neutrinos from more distant sources; it simply meant that we could not reliably match them to any known source in the sky. Although neutrinos react very weakly with matter, the probability of a reaction increases with an increase in their energy.
And here comes the IceCube observatory .

The IceCube Observatory, the first neutrino observatory of its kind, is designed to observe these elusive particles of high energy under the ice of Antarctica.
Deep under the ice of the South Pole, inside the IceCube is a cubic kilometer of solid matter that helps to find these almost massless neutrinos. When neutrinos pass through the Earth there is always a chance that they will react with the particle there. The interaction will lead to the appearance of a shower of particles, which should leave unequivocal traces on the detectors.

An illustration of how a neutrino reacted with an ice molecule, producing a secondary particle - a muon - moving in ice at a relativistic speed, and leaving a trail of blue light behind it
In six years of operation, IceCube detectors detected more than 80 high-energy cosmic neutrinos with energies of more than 100 TeV: this is more than 10 times higher than the highest energy values achieved by any particles at the Large Hadron Collider. Some of them even approached the scale in PeV, reaching energies a thousand times larger than those necessary to create even the heaviest of the known fundamental particles.
Despite all these neutrinos from space that arrived on Earth, we have so far not been able to compare them with a source in the sky, the location of which we would know for sure. Detection of these neutrinos is an amazing achievement, but we cannot understand which process generates them, unless we can match them in a real, observable object of the Universe — for example, one that we can also observe in electromagnetic radiation.

When a neutrino interacts with particles of pure Antarctic ice, it generates secondary particles, leaving a trace in the form of blue light during the passage through the IceCube detector
. Theorists had no problems with coming up with ideas, for example:
- hypernovae , the brightest varieties of supernovae,
- gamma bursts,
- flashes generated by black holes
- quasars, the largest of the active black holes of the universe.
But to solve this question, evidence was needed.
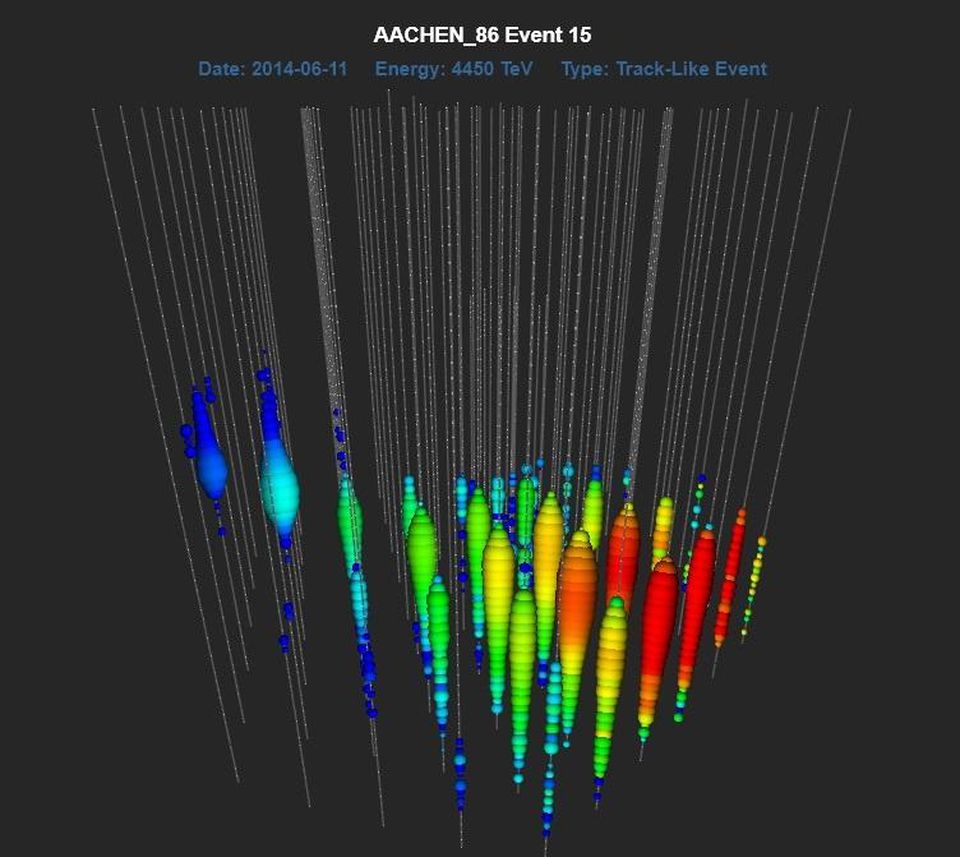
An example of a high-energy neutrino detected on the IceCube: a 4.45 PeV neutrino collided with a detector in 2014.
IceCube monitored and released reports after each ultra-high energy neutrino detected by them. On September 22, 2017, another such phenomenon was observed: IceCube-170922A. In the report, scientists announced the following:
On September 22, 2017, IceCube discovered a very high energy event with a high probability having an astrophysical source. This event was detected by the Extremely High Energy (EHE) high energy event tracking system. IceCube was in normal operation. EHE events are usually associated with an interaction peak outside the detector where a muon is generated that crosses the detector volume with a high level [energy].

Cosmic rays cause air showers of particles, colliding with protons and atoms of the atmosphere, and emit light due to Cherenkov radiation. Observing cosmic rays in the sky and neutrinos reaching the Earth, we use coincidences to uncover the sources of both phenomena.
This enterprise is interesting not only by neutrino particles, but also by cosmic rays as a whole. Despite the fact that we have seen millions of high-energy cosmic rays over the past hundred years, we do not know the sources of most of them. And this is true for protons, nuclei and neutrinos, both created at their source and resulting from air showers.
That is why it was so interesting that, in addition to a warning about the phenomenon, IceCube also gave out the heavenly coordinates from where these neutrinos were supposed to arrive:
- RA: 77.43 degrees (-0.80 degrees / + 1.30 degrees 90% PSF containment) J2000
- Dec: 5.72 degrees (-0.40 degrees / + 0.70 degrees 90% PSF containment) J2000
And they led observers who were trying to make subsequent observations in the electromagnetic spectrum to this object.
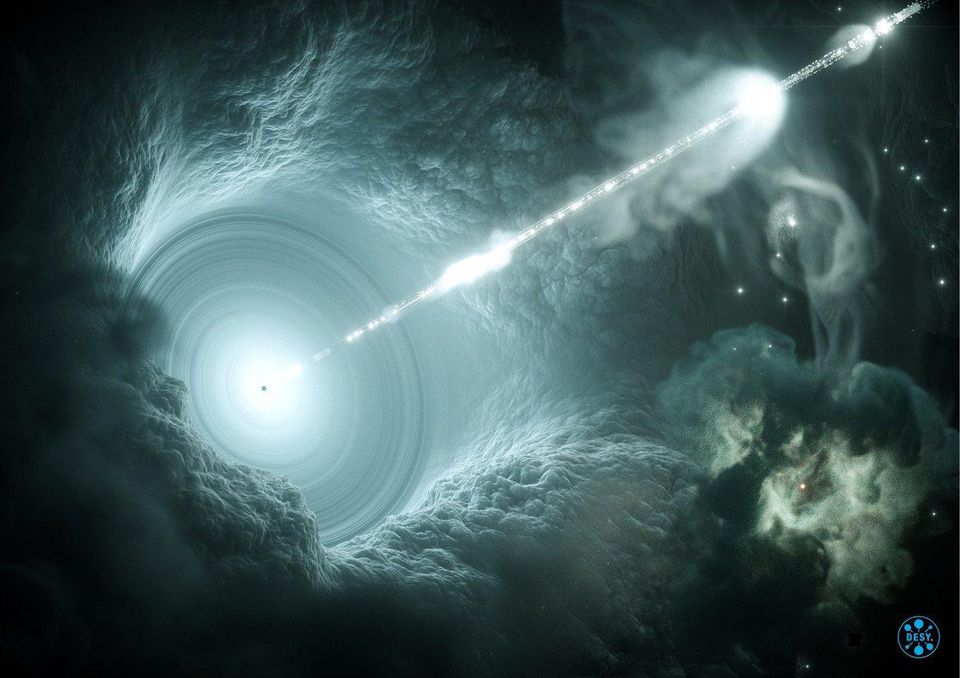
Artistic image of the active galaxy nucleus. A supermassive black hole in the center of an accretion disk sends narrow jets of high energy matter into space, perpendicular to the disk. Blazar, located 4 billion light years away, has become the source of these cosmic rays and neutrinos.
It turned out to be a blazar: a supermassive black hole in the active state, feeding on matter and accelerating it to incredible speeds. Blazars are the same quasars, but with one important difference. Quasars can be oriented in any direction, and at Blazar one of the jets is always directed directly to the Earth. They are called blazars from the word blaze, which means "shine, burn brightly" - they shine straight in our direction.
This particular blazar is known as TXS 0506 + 056 , and many observatories, including the Fermi Observatory at NASA and the MAGIC ground telescope in the Canary Islands, immediately detected the gamma rays emanating from it. About 20 observatories on Earth and in space conducted observations on the traces of neutrino detection using the location given by the IceCube detector last September. This made it possible to determine what scientists believe is the source of high-energy neutrinos, and, therefore, cosmic rays. In addition to neutrinos, observations made across the entire electromagnetic spectrum were made in the gamma, x-ray, optical, and radio frequency ranges.

In addition, upon arrival of the neutrino, it turned out that the blazar is in a radiant state corresponding to the most active particle ejection for such objects. Since particle ejection has its highs and lows, researchers from IceCube combed records for ten years of observations that preceded the outbreak of September 22, 2017, in search of any events related to neutrinos that could have come from TXS 0506 + 056 .
They immediately discovered that neutrinos came from this object in several flashes, which stretched for many years. By combining observations of neutrinos with observations in the electromagnetic range, we were able to reliably conclude that high-energy neutrinos come to us from blazars, and that we have the ability to detect them, even from such great distances. TXS 0506 + 056is 4 billion light years away from us.

Blazar TXS 0506 + 056 is the first confirmed source of high-energy neutrinos and cosmic rays. The picture shows the location of the blazar, located in the sky at the left shoulder of the constellation Orion.
From a single observation of an event with several "messengers" you can derive a huge amount of information:
- It has been demonstrated that blazars are at least one of the sources of cosmic rays.
- The appearance of neutrinos requires decaying pions that appear due to rapidly moving protons.
- The first convincing evidence of the acceleration of protons by black holes.
- TXS 0506 + 056 is one of the brightest sources of the Universe.
- Associated gamma rays suggest that cosmic neutrinos and cosmic rays, at least from time to time, may have a common source.

Cosmic rays emitted by astrophysical sources of high energy, can reach the Earth's surface. When a cosmic ray collides with a particle of the earth's atmosphere, it creates a shower of particles, which we can detect using arrays of ground-based sensors. Finally, we have disclosed their main source.
According to Francis Hulsen, IceCube neutrino observatory research leader:
Interestingly, in the astrophysical community, it was widely believed that blazars can hardly be considered sources of cosmic rays - and here it is ... The ability to build telescopes from all over the world and make discoveries at many wavelengths together with a neutrino detector like IceCube, marks a new milestone in Scientists call multi-messenger astronomy astronomy.
The era of astronomy of several messengers has officially come, and now we have three completely independent, complementary ways to look at the sky: with the help of light, neutrinos and gravitational waves. We have learned that blazars, once thought to be unlikely candidates for generating high-energy neutrinos and cosmic rays, actually produce both.

The artist's idea of the remote quasar 3C 279. Outgoing from both poles of the jet is a common phenomenon, but it very rarely happens that such a jet points directly to us. When this happens, we get the blazar - as now confirmed, the source of cosmic rays and high-energy neutrinos that we have observed for many years.
Together with this discovery, a new scientific field, the astronomy of high-energy neutrinos, is officially launched. Neutrinos are no longer a byproduct of other interactions or a space wonder, barely extending beyond the limits of the solar system. Now we can use them as a fundamental probe of the Universe and the laws of physics themselves. One of the main goals of the IceCube was to identify sources of high-energy cosmic neutrinos. With the confirmation of Blazar TXS 0506 + 056 as a source of both these neutrinos and cosmic rays, one of many of the cosmic dreams finally came true.