Ask Ethan: how fast do gravitational waves move?
- Transfer

One of the most unexpected predictions of Einstein's General Theory of Relativity is the existence of not only matter, radiation and other forms of energy based on particles, but also the existence of gravitational radiation itself, a fundamental ripple on the fabric of space-time. This is one of the most difficult to understand concepts, and our reader wants to learn more on this topic:
Gravitational waves are perturbations of space-time traveling with speed with. However, space-time can expand and contract faster with. But expansion, followed by contraction, is essentially the definition of a compression wave. It seems like a paradox: gravitational waves move with speed c, but for them there seems to be a possibility of superluminal movement. How to solve it?
To begin, let us begin with the concept of this radiation, and how it appears.

In electromagnetism, even in classical, there are only two things necessary for the appearance of electromagnetic radiation: the charge and the field through which it moves. An electric charge can be positive (like a proton) and negative (like an electron), and if it moves in a magnetic field, it will accelerate it, and spin it, sending it on a cyclic or spiral trajectory.
The stronger the field, the higher the speed, and the greater the charge-to-mass ratio, the greater the acceleration (or change in motion).
But in such interactions energy and momentum must be conserved, therefore, in electromagnetism, each time the charge is accelerated under the action of an external field, it has to emit radiation. It manifests itself in the form of photons, and is called, depending on the mode of occurrence, bremsstrahl (German Bremsstrahlung), cyclotron or synchrotron radiation.
There would be no gravitational radiation in Newtonian physics, but Einstein GTR changes everything. Massive sources, such as particles, have an analogue of a gravitational charge, and curved space is an analogue of a gravitational field. Every time a massive particle moves in a curved space, and it can be severely curved near a star, a white dwarf, a neutron star, or a black hole, it will emit an analog of electromagnetic radiation: gravitational radiation.

This new form of radiation will not be a photon or some other type of radiation, but a gravitational wave propagating in the very fabric of space. For a mass of the order of the Earth rotating around the Sun, the gravitational radiation turns out to be so small that an appreciable change in the orbit should take about 10140 ages of the universe. We will never notice. But for systems with larger masses, shorter distances and stronger fields, the consequences are more serious: for double pulsars, orbits around a supermassive black hole at the center of our Galaxy or merging black holes. In such cases, a decrease in the orbit can be observed, and because of the need to save energy, we know that something is taking it away.
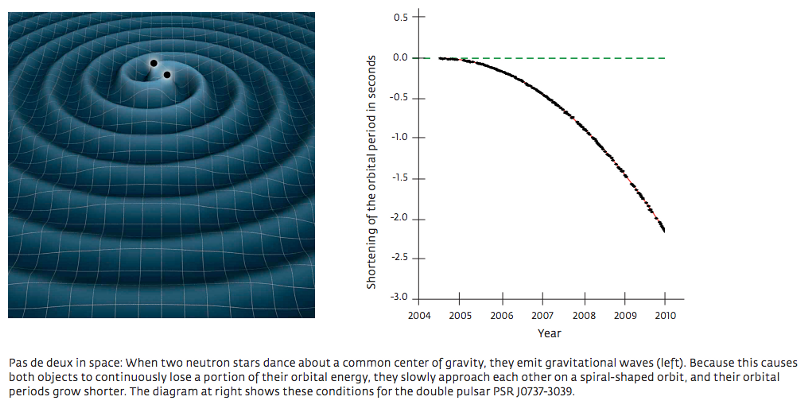
This should be gravitational radiation (it is also gravitational waves), and thanks to the observation of double pulsars we know that the speed of this radiation must be equal to the speed of light with an accuracy of 0.2%! In other words, the waves move through space at the same speed as the photons. The main difference is that in the case of gravitational radiation, these waves are an integral part of the fabric of space itself.

So what happens if these waves, as described in the reader's question, are not created in static space, but in an expanding Universe? They stretch and expand just like photons.
The wavelength of a photon traveling in an expanding universe, stretches along with the expansion of space. The concentration and energy of these particles decrease, and although they always propagate at the speed of light, the distances between the source and the observer increase. For example, at the very beginning of the hot Big Bang, 13.8 billion years ago, only 10 -33 seconds after inflation:
• The photon that reached us today would be just 100 meters from us 13.8 billion years ago.
• It would travel 13.8 billion years, and travel 13.8 billion light years through an expanding universe, and its wavelength would increase by 28 orders of magnitude.
• The source of the photon that reached us today would have been 46.1 billion light years away.
Sounds crazy? This same madness happens with gravitational waves! Gravitational ripples should also spread over the expanding Universe, also move in space at the speed of light (whether it expands or not), and its wavelength stretches in the same way as photons do. Gravitational waves "ride" on the fabric of space just as water "ride" on the surface of the water. If you drop a stone in a river, the ripples will not just spread outward along the radius. It will spread outward, and at the same time it will be carried away by the flow of the river.

Gravitational ripples on the fabric of space behave in the same way: waves move as fast as they always move in the medium — at the speed of light from — but sometimes the environment also moves. This does not mean that they exceed the speed of light, just as photons do not exceed it when they are 46 billion light years from the source from which they began their journey 13.8 billion years ago. Gravitational waves behave as they should. Analogue with compression and expansion is really very good. A passing wave distorts the fabric of space, and all things and particles in it, stretching and squeezing them in a certain way.
But it spreads through the Universe at the speed of light and taking into account how the fabric of space itself behaves: it expands, contracts, or remains static. This is the resolution of the paradox: the waves travel at a speed of c, regardless of what happens to the medium through which they pass!