Look into the future to understand the past.
- Transfer
An alternative to the Newtonian view of the world promises to explain the oddities of quantum physics.

Judging by the best of physical theories, you misunderstand the time. In Einstein's GR, there is no difference between the past and the future, not to mention the concept of "now." Also, there is no direction in which time flows; instead, space and time simply exist in some four-dimensional structure. Moreover, all the fundamental laws of physics work in the same way, both forward and backward.
These facts are not easy to accept, since they contradict our subjective perception of time. But do not be discouraged: it is not easy to accept them even to physicists, and this tension leads physics into conflict not only with common sense, but also with itself. And although physicists talk a lot about the symmetry of time, they allow themselves to seek the causes of phenomena occurring in the world, only in the past, not in the future.
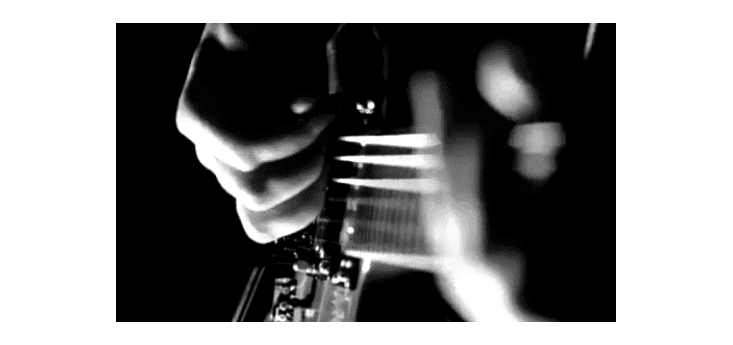
Just as the fastening of the ends of a guitar string determines its vibrations, the distant past and the distant future of the Universe can affect today's events.
When formulating explanations, most of us think in terms of Isaac Newton, established more than 300 years ago. This "Newtonian mechanics" takes the past as a basis and uses it to solve the future, explaining our Universe step by step. Some researchers even think of the universe as the output of a computer program. Such a picture is a natural consequence of this scheme. Even after our concept of time has changed in the last century, Newtonian mechanics somehow remained in the most popular physical systems of opinion.
But using the old Newtonian system of thinking on new effects of a quantum scale, we found ourselves in situations that cannot be explained. If these phenomena are inexplicable, then maybe we are simply presenting them incorrectly. The best explanations become available, if only we are willing to take into account also the future. But Newtonian mechanics are initially incapable of such timeless explanations. Computer programs run in one direction and attempts to combine two programs running in opposite directions lead to a quagmire of paradoxes characteristic of poorly designed time travel films. To consider the future with the same seriousness as the past, we clearly need an alternative to Newtonian mechanics.
And we have it. Most physicists are aware of the existence of another belief system, an alternative in which space and time are analyzed impartially. This so-called. Lagrangian mechanics are also ancient roots, and it has become an indispensable tool in all areas of fundamental physics. But even physicists using this approach oppose the last obvious step: to present Lagrangian mechanics not as a mathematical trick, but as a way of explaining the world. Perhaps we are not taking our own theories seriously enough.
Lagrangian mechanics not only provide explanations based on the future. She demands them. Equally considering the past and the future, this mechanics avoids paradoxes and makes possible new methods of explanations. And it may be exactly the point of view that is required for the next breakthrough.
The first step to understanding Lagrangian mechanics is to completely withdraw from the flow of time from Newtonian. It is easier to do this, considering the regions of space-time holistic: considering the entire length at once, and not as successive frames of the film. We can depict space-time in the form of fixed four-dimensional structures not only with spatial, but also with time frames - the initial and final borders of the region.
All classical physics, from electricity to black holes, can be expressed through the simple Lagrangian principle of minimizing action. To use it on a segment of space-time, you first describe the limitations of physical parameters throughout its length. Then for each set of possible events in this framework, you calculate the value of the “action”. The set of events with the smallest magnitude of the action is the one that happens, taking into account the initial restrictions and a few technical points.
For example, for a beam of light traveling from point A to point B, the action corresponds to the duration of the travel time. The path will be the fastest, given the obstacles. If this is the case, then, the beam of light bends on the surface of the glass to minimize travel time. Lagrangian mechanics in quantum physics works a little differently and leads to the appearance of probabilities rather than exact answers, but the essence is the same: space-time constraints are imposed simultaneously.
By Newton's logic, this is strange. A ray of light at point A somehow foresees the future (about point B and future obstacles), has extensive computational capabilities (to count several ways) and knowledge (to choose the smallest). But oddity is only proof that Newtonian and Lagrangian thinking does not mix - and that we probably do not need to attribute human traits to light.
Instead of explaining events only on the basis of the past, Lagrangian mechanics begins with general boundary constraints - including the final one. If you do not impose a final restriction — for a ray of light this is point B — this approach will not produce the correct answer. But if used correctly, the success of mathematics proves the logical priority of boundary constraints - the border of any region of space-time explains its contents.
Langrange's approach provides the most elegant and flexible description of physics, and physicists often prefer it. But, despite the applicability of these principles, even physicists using them do not take them literally. It is difficult to accept that events can be explained on the basis of what is happening in the future. After all, there are obvious differences between the past and the future. And if the arrow of time is so obvious to us, how can future restrictions affect what is happening on a par with the past?
But there is a way to reconcile Lagrangian mechanics with our everyday experience. We only need to think broadly enough, without losing sight of the details.
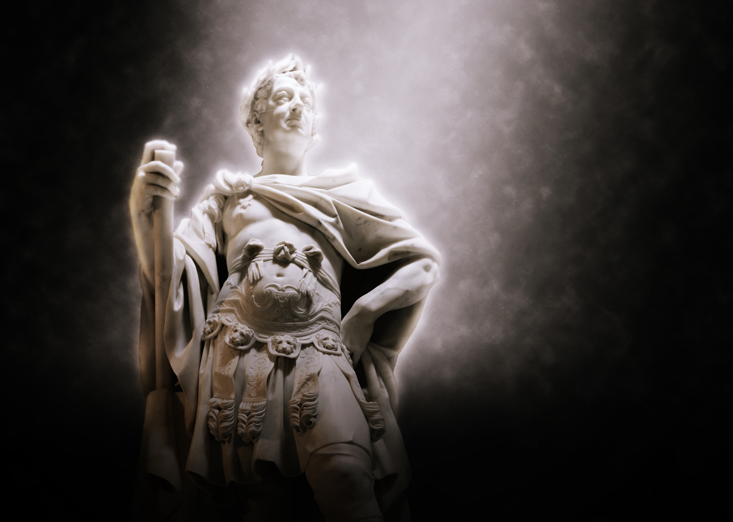
Physics is reversible in time. If the spotlight illuminates the statue, you can also say that the statue illuminates the spotlight. Usually we do not do this, because it violates our expectations about the meaning of the word "explanation."
Suppose you photographed a statue with a flash. Each ray of light obeys the principle of least action, producing a perfectly symmetrical path in time. But together they show an obvious asymmetry: the initial constraints of A are gathered together in a flash, and the final constraints are distributed across the statue. Moreover, it is obvious that the propagation of light from A is a much better explanation for the illumination of B, rather than vice versa. Even if we looked at the paths of the rays of light in the opposite direction, no one could argue that the light is concentrated in a flash due to the complex light patterns on the statue.
From here it can be taken out that satisfactory explanations manage with complex events by simple methods. They take one fact with several relevant parameters to explain a variety of phenomena. This should be obvious regardless of the mechanics used.
But this asymmetry of A and B does not refute the Lagrangian view, which only postulates that A and B together best explain the details of what happened between them. Even in Lagrangian mechanics, A and B are interdependent. To trace their connection, let's look more widely. According to the principle of constraints of Lagrangian mechanics, explanations are not lined up in a chain, but are invested one into the other. In other words, we do not say that event A led to event B, which led to event B. We work with a small region of space-time entirely; then we consider it as part of a larger region (in space and time). Applying the same Lagrangian logic, we get that larger boundaries should explain everything within them, including the original boundaries.
By running this procedure for the statue, we find the same asymmetry of the light bulb and the statue on an enlarged scale. That is, we are satisfied with the description of the camera flash in the past, but we do not explain the highlight of the statue, looking into the future. Then we enclose this system into an even larger one, and so on, until we reach the cosmological boundaries — the outer boundaries of the Universe. As far as we know, the same asymmetry is observed on such scales - an unusually smooth distribution of matter during the Big Bang and a more erratic distribution in the future.
If we consider ordinary regions of space-time from a Lagrangian point of view, the fact that the initial boundaries (rays of light emanating from the flash lamp) are simpler than the final (illuminated statues) proves that our nearest cosmological boundary is in the past. This sequence implies that in a comparable future there are no corresponding cosmological boundaries. And if you take the Big Bang as the best explanation for the obvious properties of the Universe, the obvious direction of the flow of time does not differ from the spatial temperature gradient that you feel standing at the cold window. In none of these cases will space or time be asymmetrical; it's just a matter of your location relative to the nearest border.
On the classical scale, which we usually encounter, we will not receive new information from the future border compared to the border in the past. If this were true on all scales, Lagrangian mechanics would have problems, because the future boundary would have no effect whatsoever. But it will not be so if we sink to the level of quantum uncertainty: the details of the microscopic future cannot be inferred from the past. It is on quantum scales that all the possibilities of Lagrangian mechanics become apparent.
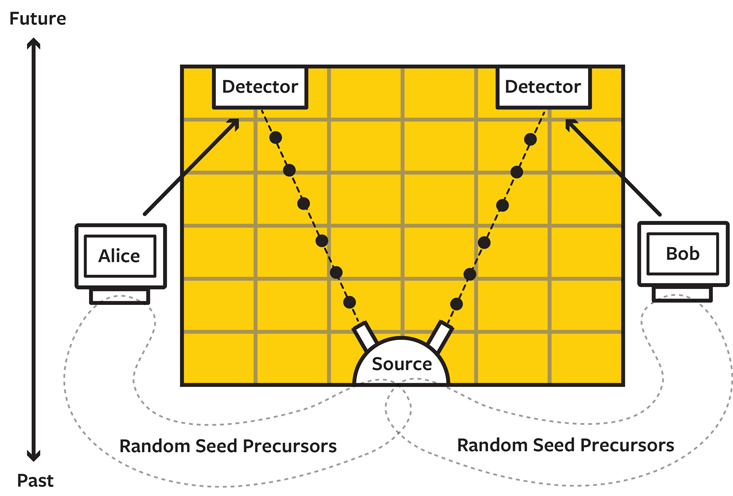
Intricate history: in a standard quantum entanglement experiment, pairs of particles fly out of the source and are measured by detectors. Two computers, Alice and Bob, generate random numbers to monitor the detectors. Events on the detectors control what happens at the source, despite the fact that they are located in the future.
Quantum entanglement is a concept that rejects Newtonian mechanics. Details are not important for us, so we consider the general essence of a typical experiment on entanglement. The device in the center creates two particles. The left one goes to the detector, computer-controlled Alice, and the right one - to the remote detector, controlled by the computer Bob. Detectors measure their particles in one of several ways determined by random numbers. As demonstrated in the famous experiment of the Irish physicist John Bell in the 60s, the measurement results correlate in a way that defies ordinary explanation.
Specifically, the total particle past is not enough to explain the measured correlations, at least not for the full range of measurements that Alice and Bob can choose at random. Of course, many scientists want to explain this from a physical point of view and they don’t like trying to do it through pure mathematics. Remaining in misunderstandings, they begin to cause mysterious entities that do not really exist in space or time (and themselves require explanation) or even travel faster than light (breaking everything we know about Einstein's theory of relativity).
If we discard these desperate attempts, then everyone will agree that a natural explanation could be found only if the particles could predict the random settings of Alice and Bob beforehand. But most of the proposals for the transfer of particles of this information look even more desperate, even a scam: the particles allegedly somehow sniff out all the input data of random number generators and use this information to predict the future settings of the detectors.
Almost no one considers this to be a worthy explanation of experiments on entanglement, as you would not accept the explanation of the localization of light on the flash lamp, reflected from the complex forms of the statue. Such attempts violate our reasonable standards of explanation: the intended mechanism of work is much more complicated than the simple results that he tries to explain.
In the example with the statue, the obvious solution would be to find simpler bounds - flares - for the best explanation. For quantum entanglement, considered from the point of view of Lagrangian mechanics, a reasonable explanation will be almost as obvious. It does not affect the complex preliminary work with the detector settings, it lies in the future detector settings.
Mysterious entangled particles exist in the foggy region of space-time in the future and the boundaries of this region include their preparation and their detection by the detector. The settings chosen by Alice and Bob are physically implemented in detectors on the final boundary - exactly where the Lagrangian mechanics tells us to look for explanations. We only need to allow the particles to confine themselves to this boundary in the future, and a simple explanation of entanglement experiments immediately appears. In this case, the future together with the past can best explain the observations.
Quantum entanglement may not be the only mystery that can be solved by seriously considering the future as an explanation. In other quantum phenomena, a simpler explanation may also be found, operating in simple space and time without any actions at a distance. It is possible that the probabilities in quantum theory will turn out to be the same as the probabilities in any other scientific field: existing due to unknown parameters (since some of them will be in the future).
Such a study will raise important questions. If the future can impose restrictions on the past, why are the consequences limited to the quantum level? Why can't we use quantum phenomena to send messages to the past? On what scale are space constraints dominated and how can Lagrangian approaches be generalized so that it all works?
Finding answers to them can not only help physics, but also better understand how we fit into our four-dimensional Universe. For example, according to Lagrangian mechanics, the microscopic details of any region are not completely limited by past boundaries. At the level of brain atoms, there are important but unknown limitations in the future. Perhaps these arguments will even help to understand free will, justifying how the future is not fully determined by the past. This will require us to rethink the idea of the existence of a simple and obvious difference between a fixed past and an open future.
Almost every time science found a deeper, simpler and more satisfactory explanation, it led to a cascade of other scientific discoveries. Therefore, if there is a deeper level of quantum phenomena that we have not yet mastered, its development will lead to critical breakthroughs in a large number of technologies that use quantum effects. Erroneous instincts hindered scientific progress in the past, and our instincts relating to time are perhaps one of the most powerful. But there is an understandable way to explain the deepest secrets of nature, if only we can force ourselves to look into the future.