
Transistor History, Part 3: Multiple Reinvention
- Transfer

Other articles in the series:
- Relay history
- The history of electronic computers
- Transistor history
- Internet history
For more than a hundred years, an analog dog has been wagging its digital tail. Attempts to expand the capabilities of our senses - sight, hearing, and even, in a sense, touch, led engineers and scientists to search for the best components for telegraph, telephone, radio, and radar. By sheer luck, these searches found a way to create new types of digital machines. And I decided to tell the story of this constant exaltation , during which telecommunication engineers supplied the source materials for the first digital computers, and sometimes even designed and created these computers themselves.
But by the 1960s, this fruitful collaboration came to an end, and with it my story. Manufacturers of digital equipment no longer needed to look into the world of telegraph, telephone and radio in search of new, improved switches, since the transistor itself provided an inexhaustible source of improvements. Year after year, they dug deeper and deeper, always finding ways to exponentially increase speed and reduce cost.
However, none of this would have happened if the invention of the transistor had stopped at the work of Bardin and Brettein .
Slow start
There was no active enthusiasm in the popular press for Bell's announcement of the invention of the transistor. On July 1, 1948, The New York Times took three paragraphs to this event at the bottom of the Radio News summary. Moreover, this news appeared after others, obviously considered more important: for example, the one-hour radio show “Waltz Time”, which was supposed to appear on NBC. In hindsight, we might want to laugh, or even scold unknown authors - how could they not recognize the event that turned the world upside down?

But a look into the past distorts perception, amplifying those signals whose significance we know, although at that time they were lost in a sea of noise. The 1948 transistor was very different from the transistors of computers, on one of which you read this article (if you did not decide to print it). They differed so much that, despite the same name, and the continuous line of inheritance connecting them, they should be considered different species, if not different genera. They have different compositions, different structures, different principles of functioning, not to mention the gigantic difference in sizes. Only thanks to constant repeated inventions, the awkward device constructed by Bardin and Brettin could transform the world and our lives.
In fact, a germanium transistor with a single contact point did not deserve more attention than it received. He had several defects inherited from the electron tube. He, of course, was much smaller than the most compact lamps. The absence of a hot thread meant that it produces less heat, consumes less energy, does not burn out and does not require heating before use.
However, the accumulation of dirt on the contact surface led to failures and nullified the potential for a longer service life; he gave a noisier signal; worked only at low power and in a narrow frequency range; refused in the presence of heat, cold or humidity; and it couldn’t be produced uniformly. Several transistors created in the same way by the same people would have defiantly different electrical characteristics. And all this was accompanied by a cost eight times greater than that of a standard lamp.
Only by 1952 did Bell's laboratories (and other patent owners) solve production problems enough for transistors with a single point of contact to become practical devices, and even then they did not particularly spread beyond the hearing aid market, where price sensitivity was relatively low. and the advantages regarding battery life exceeded the disadvantages.
However, then the first attempts had already begun to turn the transistor into something better and more useful. They actually started much earlier than the moment when the public learned about its existence.
Shockley's ambition
By the end of 1947, Bill Shockley, in great excitement, embarked on a trip to Chicago. He had vague ideas on how to surpass the transistor recently invented by Bardin and Brettein, but so far he had no chance to develop them. Therefore, instead of enjoying a break between the stages in his work, he spent Christmas and New Year at the hotel, filling out about 20 pages of a notebook with his ideas. Among them was a proposal for a new transistor consisting of a semiconductor sandwich - a slice of p-type germanium between two n-type pieces.
Encouraged by the presence of such an ace up his sleeve, Shockley made a claim to Bardin and Bretstein on their return to Murray Hill, demanding all the glory for the invention of the transistor. Was it not his idea of a field effect that made Bardin and Brettein sit in the laboratory? Is it not necessary because of this to transfer all rights to a patent to him? However, Shockley’s trick turned out to be sideways: Bell’s patent lawyers found out that an unknown inventor, Julius Edgar Lilienfeld , patented a field effect semiconductor amplifier almost 20 years earlier, in 1930. Lilienfeld, of course, did not realize his idea, given the state of materials at that time, but the risk of intersection was too great - it was better to completely avoid mentioning the field effect in the patent.
So, although Bell’s laboratories gave Shockley a generous share of the inventor’s fame, they mentioned only Bardin and Bretstein in the patent. However, you can’t do what you did: Shockley’s ambitions destroyed his relationship with two subordinates. Bardin stopped working on the transistor, and concentrated on superconductivity. He left the laboratory in 1951. Bretstein remained there, but refused to work with Shockley again, and insisted on transferring to another group.
Due to the inability to work with other people, Shockley did not advance in the laboratories, so he also left there. In 1956, he returned home to Palo Alto to establish his own transistor manufacturing company, Shockley Semiconductor. Before leaving, he broke up with his wife Gene when she was recovering from uterine cancer, and married Emmy Lenning, whom he soon married. But of the two halves of his California dream - a new company and a new wife - only one was fulfilled. In 1957, his best engineers, enraged by his management style and the direction in which he led the company, left him to found a new company, Fairchild Semiconductor.
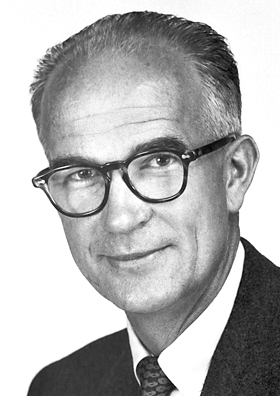
Shockley in 1956
So Shockley threw the empty shell of his company and got a job at the Department of Electrical Engineering at Stanford. There, he continued to push his colleagues (and his oldest friend, physicist Fred Seitz ) away from him with theories of racial degeneration and racial hygiene that had interested him , topics that were unpopular in the United States since the last war, especially in academia. He found pleasure in unleashing disputes, inflating the media and provoking protests. He died in 1989, moving away from children and colleagues, and was visited only by his second wife, forever devoted to him, Emmy.
Although his pathetic attempts in the field of entrepreneurship failed, Shockley dropped the grain into fertile soil. The San Francisco Bay Area has produced many small electronics companies, which were spiced up by federal government funding during the war. Fairchild Semiconductor, a random offspring of Shockley, spawned dozens of new firms, a couple of which are known today: Intel and Advanced Micro Devices (AMD). By the early 1970s, the area earned the mocking nickname of Silicon Valley. But wait a minute - after all, Bardin and Brettein created a germanium transistor. Where did silicon come from?

So in 2009, it looked like an abandoned place in Mountain View, where Shockley Semiconductor was previously located. Today the building is demolished.
To Silicon Crossroads
The fate of a new type of transistor, invented by Shockley in a Chicago hotel, was much happier than that of his inventor. All thanks to the desire of one person to grow single pure semiconductor crystals. Gordon Thiel, a physical chemist from Texas who studied then useless germanium for his doctorate, got a job in Bell's laboratory in the 1930s. Learning about the transistor, he was convinced that its reliability and power can be significantly improved by creating it from a pure single crystal, and not from the polycrystalline mixtures then used. Shockley rejected his attempts, considering them a waste of resources.
However, Teal persisted and achieved success, with the help of mechanical engineer John Little, creating an apparatus that takes out a tiny crystal nucleus from molten germanium. Cooling around the nucleus, germanium expanded its crystal structure, creating a continuous and almost pure semiconducting lattice. By the spring of 1949, Thiel and Little could create crystals on request, and tests showed that they left far behind their polycrystalline competitors. In particular, minor carriers added to them could survive within a hundred microseconds or even longer (against no more than ten microseconds in other crystal samples).
Now Teal could afford more resources, and recruited more people to his team, among whom was another physical chemist who came to Bell's laboratory from Texas - Morgan Sparks. They began to change the melt for the manufacture of p-type or n-type germanium, adding balls of the corresponding impurities. Within a year, they improved the technology to such an extent that they could grow a germanium npn sandwich directly in the melt. And it worked exactly as Shockley predicted: the electrical signal of the p-type material modulated the electric current between two conductors connected to the n-type pieces surrounding it.

Morgan Sparks and Gordon Teal at a workbench in Bell's labs
This transistor with a grown junction surpassed its ancestor with one point contact in almost all respects. In particular, it became more reliable and predictable, produced much less noise (and, therefore, was more sensitive), and extremely energy-efficient - consuming a million times less energy than a typical electronic lamp. In July 1951, Bell's laboratories organized another press conference to announce a new invention. Even before the first transistor managed to enter the market, it, in fact, had already become insignificant.
And yet this was only the beginning. In 1952, General Electric (GE) announced the development of a new process for creating transistors with a junction, alloy method. In its framework, two indium balls (p-type donor) were fused on both sides of a thin slice of n-type germanium. This process was simpler and cheaper than growing transitions in the alloy, such a transistor gave less resistance and supported high frequencies.

Grown and Alloy Transistors
The following year, Gordon Thiel decided to return to his home state, and got a job at Texas Instruments (TI) in Dallas. The company was founded under the name Geophysical Services, Inc., and first produced equipment for oil exploration, TI opened an electronics division during the war, and now entered the transistor market under a license from Western Electric (Bell Labs manufacturing division).
Teal brought with him new skills gained in laboratories: the ability to grow and alloysilicon single crystals. The most obvious weakness of Germany was its sensitivity to temperature. When exposed to heat, germanium atoms in the crystal quickly released free electrons, and it increasingly turned into a conductor. At a temperature of 77 ° C, he generally stopped working like a transistor. The main goal of transistor sales was the armed forces - a potential consumer with low price sensitivity and a huge need for stable, reliable and compact electronic components. However, temperature-sensitive germanium would not be useful in many cases of military use, especially in the aerospace field.
Silicon was much more stable, but had to pay a much higher melting point, comparable to the melting point of steel. This caused great difficulties, given that to create high-quality transistors, very pure crystals were required. Hot molten silicon would absorb impurities from any crucible it was in. Teal and the TI team were able to overcome these difficulties with DuPont's ultra-pure silicon samples. In May 1954, at a conference at the Radio Engineering Institute in Dayton, Ohio, Thiel demonstrated that the new silicon devices manufactured in his laboratory continued to work, even when immersed in hot oil.
Successful Upstarts
Finally, about seven years after the first invention of the transistor, it could be made from material with which it became synonymous. And about the same amount of time will pass before the appearance of transistors, roughly resembling the form that is used in our microprocessors and memory chips.
In 1955, scientists from Bell's laboratories successfully learned how to make silicon transistors with a new alloying technology - instead of adding solid impurity balls to the liquid melt, they introduced gaseous additives into the solid surface of the semiconductor ( thermal diffusion) By carefully controlling the temperature, pressure and duration of the procedure, they reached exactly the required depth and degree of alloying. Strengthening control over the production process has given greater control over the electrical properties of the final product. More importantly, thermal diffusion made it possible to produce the product in batches - it was possible to alloy a large silicon plate, and then cut it into transistors. The military secured funding for Bell's laboratories, since the organization of production required high upfront costs. They needed a new product for an ultra-high-frequency line early warning radar detection (" Dew line"), a chain of Arctic radar stations designed to detect Soviet bombers flying from the North Pole, and they were ready to put out $ 100 per transistor (these were the times when a new car could be bought for $ 2000).
Doping along with photolithography , which controlled by the arrangement of impurities, they opened up the possibility of etching the entire circuit entirely on a single semiconductor substrate - this was simultaneously thought up by Fairchild Semiconductor and Texas Instruments in 1959. Fairchild's “ planar technology ” used a chemical the deposition of metal films connecting the electrical contacts of the transistor eliminated the need for manual wiring, reduced production costs and increased reliability.
Finally, in 1960, two engineers from Bell's laboratories (John Atalla and Devon Kahn) implemented the original field effect Shockley transistor concept. A thin oxide layer on the surface of the semiconductor was able to effectively suppress surface states, as a result of which the electric field from the aluminum gate penetrated into the silicon. Thus was born the MOSFET [metal-oxide semiconductor field-effect transistor] (or MOS structure, from metal-oxide-semiconductor), which turned out to be so easy to miniaturize, and which is still used in almost all modern computers (it’s interesting that Atalla was originally from Egypt, and Kang from South Korea, and almost only these two engineers from our entire history have no European roots).
Finally, thirteen years after the invention of the first transistor, something appeared that looked like the transistor of your computer. It was easier to produce, it used less energy than a planar transistor, but it reacted rather slowly to the signals. Only after the spread of large integrated circuits with hundreds or thousands of components located on a single chip did the advantages of field effect transistors come to the fore.

Illustration of a patent for a field effect transistor
The field effect was Bell's last major contribution to the development of the transistor. Major electronics manufacturers such as Bella Labs (with their Western Electric), General Electric, Sylvania, and Westinghouse have built up an impressive body of research on semiconductors. From 1952 to 1965, only Bell's laboratories registered more than two hundred patents on this subject. Still, the commercial market quickly fell into the hands of new players such as Texas Instruments, Transitron and Fairchild.
The early transistor market was too small for major players to pay attention to: about $ 18 million a year in the mid-1950s, compared with a total electronics market of $ 2 billion. However, the research laboratories of these giants served as unintended training camps, where young scientists could absorb knowledge related to semiconductors, so that after they move on to selling their services to smaller firms. When the tube electronics market began to shrink seriously in the mid-1960s, it was too late for Bella, Westinghouse, and the labs to compete with upstarts.
Transition computers to transistors
In the 1950s, transistors invaded the electronics world in the four most significant areas. The first two were hearing aids and portable radios, in which low power consumption, and, as a result, long battery life, overpowered other considerations. The third was military use. The U.S. Army had high hopes for transistors as reliable and compact components that can be used anywhere from field radio to ballistic missiles. However, at first their spending on transistors was more like betting on the future of technology than on confirming their then value. And finally, there were more digital calculations.
In the computer field, the drawbacks of switches on electronic tubes were well known, and some skeptics before the war even believed that an electronic computer could not be made a practical device. When thousands of lamps were assembled in one device, they devoured electricity, generating a huge amount of heat, and in terms of reliability, one could only rely on their regular burnout. Therefore, the low-consuming, cold and threadless transistor has become the savior of computer manufacturers. Its disadvantages as an amplifier (for example, a noisier output signal) did not present such a problem when using it as a switch. The only obstacle was the cost, and in due time it would start to plummet.
All the early American experiments with transistor computers took place at the intersection of the military’s desire to explore the potential of a promising new technology, and the engineers’s desire to switch to improved switches.
In 1954, Bell’s laboratories built TRADIC for the U.S. Air Force to see if transistors would enable the installation of a digital computer on board a bomber, replacing them with analog navigation and help finding targets. MIT's Lincoln Lab developed the TX-0 computer as part of an extensive air defense project in 1956. The machine used another variant of the transistor, a surface-barrier, well suited for high-speed computing. Philco built its SOLO computer under a contract with the Navy (but really - at the request of the NSA), ending it in 1958 (using another version of a surface-barrier transistor).
In Western Europe, not so endowed with resources during the Cold War, the story was completely different. Machines like Manchester Transistor Computer, Harwell CADET(another name inspired by the ENIAC project, and encrypted by writing backwards) and the Austrian Mailüfterl were side projects using resources that their creators could scrape together - including transistors with one first-generation contact point.
There is a lot of controversy over the title of the first computer that used transistors. Everything, of course, rests on the choice of the correct definitions of words such as “first”, “transistor” and “computer”. In any case, it is known where the story ends. The commercialization of transistor computers began almost immediately. Year after year, computers for the same price became more powerful, and computers of the same power became cheaper, and this process seemed so inexorable that it was elevated to the rank of law, along with gravity and energy conservation. Do we need to argue about which pebble was the first to collapse?
Where did Moore's law come from?
Approaching the end of the switch history, it is worth asking the question: what led to the appearance of this collapse? Why does Moore's law exist (or did exist - we bet another time)? For airplanes or vacuum cleaners, there is no Moore's law, just as there is none for electronic lamps or relays.
The answer consists of two parts:
- Logical properties of the switch as an artifact category.
- The ability to use purely chemical processes for the manufacture of transistors.
First, about the essence of the switch. The properties of most artifacts are required to satisfy a wide range of inexorable physical limitations. Passenger aircraft must support the total weight of many people. A vacuum cleaner must be able to suck in a certain amount of dirt in a certain time from a certain physical area. Aircraft and vacuum cleaners will be useless if reduced to nanoscale.
The switch, an automatic switch that has never been touched by a human hand, has much less physical limitations. It should have two different states, and it should be able to inform other similar switches of a change in their states. That is, all that he should be able to do is turn on and off. What is so special about transistors? Why haven't other kinds of digital switches experienced such exponential improvements?
Here we come to the second fact. Transistors can be manufactured using chemical processes without mechanical intervention. From the very beginning, the use of chemical impurities has been a key element in transistor manufacturing. Then a planar process appeared that eliminated the last mechanical step from production - the connection of wires. As a result, he got rid of the last physical restriction on miniaturization. The transistors no longer needed to be large enough for the fingers of a person - or for any mechanical device. Everything was done by simple chemistry, on an unimaginably small scale: acid for etching, light to control which parts of the surface would resist etching, and vapors for introducing impurities and metal films onto etched paths.
And why do you need miniaturization? The reduction in size gave a whole galaxy of pleasant side effects: an increase in switching speed, a decrease in energy consumption and the cost of individual copies. These powerful incentives prompted everyone to look for ways to further reduce switches. And the semiconductor industry, over the course of a single person’s life, has gone from making switches the size of a nail to packing tens of millions of switches per square millimeter. From a request of eight dollars per switch to an offer of twenty million switches per dollar.

Intel 1103 memory chip from 1971. Individual transistors, measuring just tens of micrometers in size, are already indistinguishable by the eye. And since then they have decreased a thousand times.
What else to read:
- Ernest Bruan and Stuart MacDonald, Revolution in Miniature (1978)
- Michael Riordan and Lillian Hoddeson, Crystal Fire (1997)
- Joel Shurkin, Broken Genius (1997)