On the improvement of nuclear fuel
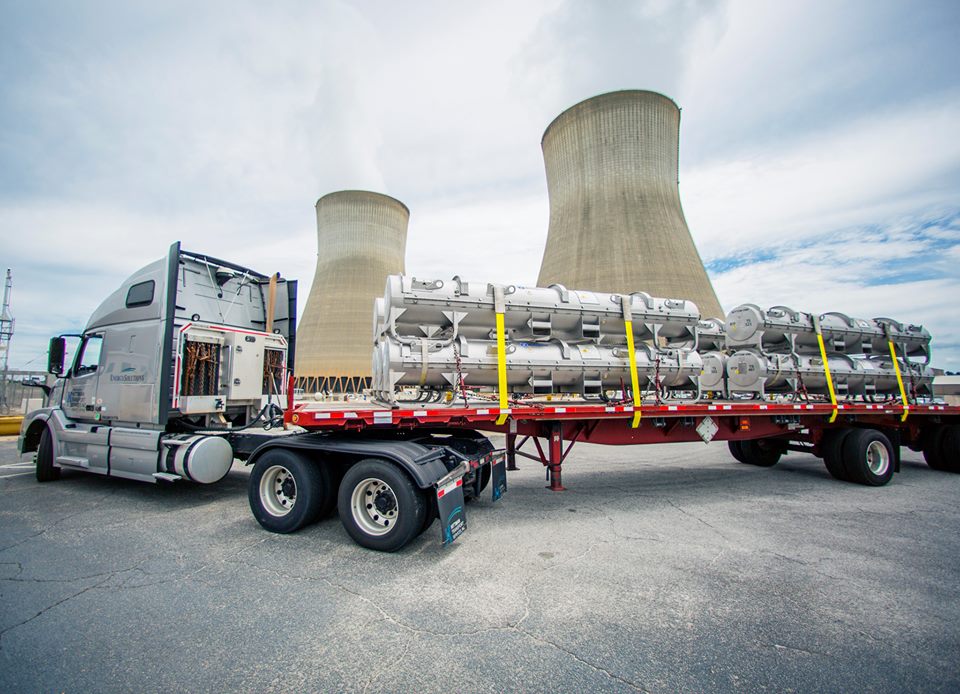
A key element in the atomic energy production system is nuclear fuel. The very, expensive high-tech product, on the creation of which work hundreds of engineers over the past 50 years. However, severe accidents, for example, at Three Mile Island and Fukushima-Daiichi NPPs, have shown that under extreme conditions, nuclear fuel is likely to fail and the accident will lead to significant consequences.
Recognizing that current fuel designs are vulnerable to severe accidents, renewed interest in alternative fuel projects, which will be more resistant to failure and hydrogen production, is the main factor leading to this failure. Such new fuel designs should be compatible with existing fuel and reactor systems and comply with all regulatory requirements of modern nuclear energy.
A little to the roots and the current situation
About 400 nuclear power units operating around the world, which provide more than 1/10 of the world's electricity, consume less fuel (by weight) in a whole year than one small coal-fired power plant does in one day.Such is the generalized statistics for understanding the energy intensity of nuclear fuel.
The current nuclear fuel or fuel assembly (FA) for most nuclear power plants is a machine-building product, which is a bundle of zirconium cylindrical shells (fuel rods) filled with enriched uranium pellets and pressurized gas. This beam is combined into a single structure with “honeycomb” type spacer grids attached to the central tube.
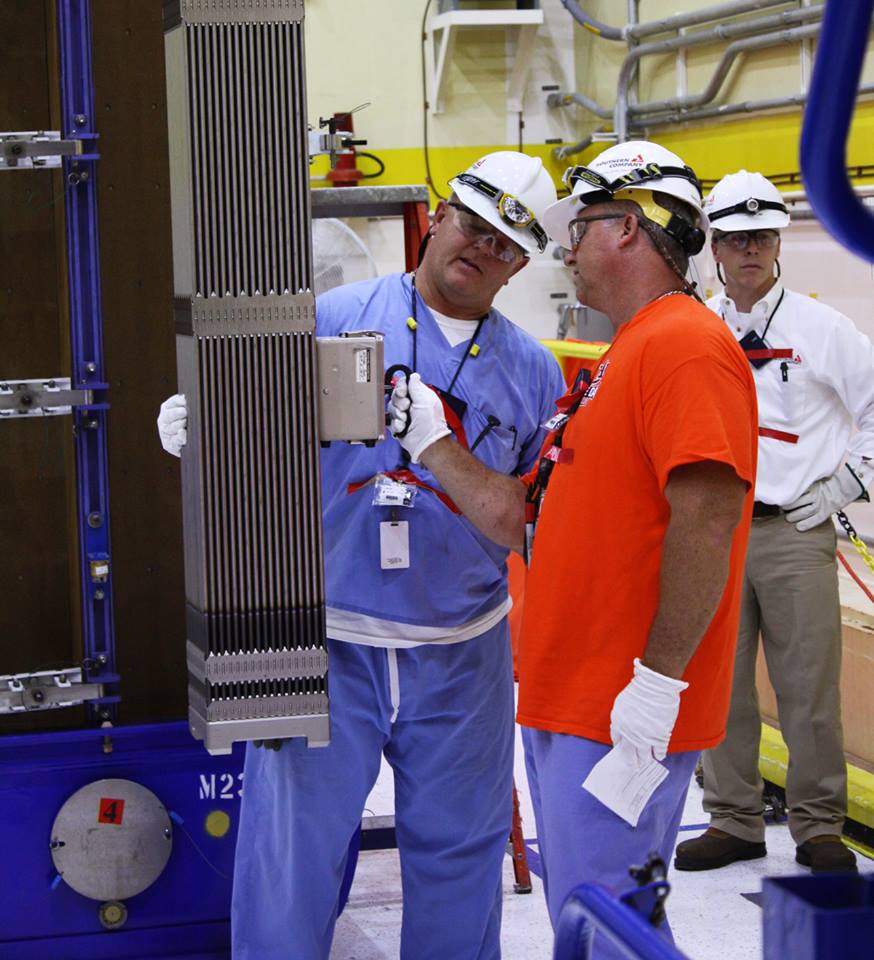
The entrance control of the “Western” fuel assembly design at nuclear power plants.
It is believed that zirconium as a fuel cell was first proposed by Admiral Hyman Rikover in June 1946 for the US Navy’s transport reactor program. This material has the necessary properties and has proven itself for a long time.
A heavy metal oxide is commonly used as a tablet material. Usually it is uranium dioxide, much less often - a mixture of oxides of uranium and plutonium. Uranium with 235U isotope content not exceeding 5% is used in modern power reactors, including uranium of natural isotopic composition (~ 0.71%) or slightly enriched.
While maintaining these common features over the past decades, there has been a gradual change in the “secondary” design features of fuel assemblies. It improved consumer properties of fuel, its reliability and safety, providing a tangible competitive advantage with early versions of fuel assemblies.
Here are some of them:
- Increasing the enrichment level: in the 1970s, it barely exceeded 3%, whereas today the maximum enrichment for light-water reactors is close to 5%. Along with the increase in the level of enrichment, it is profiled in the reactor core - up to the difference between the parts of the tablet in the advanced fuel.
- Increased uranium loading by weight. This change occurred primarily due to changes in the geometry of TVEL and structural parts of fuel assemblies. For example, for VVER reactors, the length of the “fuel” part of the assembly increased by approximately 15 cm. For western design reactors, the number of fuel elements in the square profile assembly changed: it was 15x15, it was 17x17.
- Significant changes in zirconium alloys. A striking example of the modernization of shell materials is the widespread introduction of niobium as one of the main alloying elements. In contrast to alloys that were common in the past, in which niobium was absent or contained in insignificant amounts (Zircaloy 4, Zircaloy 2), materials containing about 1% niobium began to dominate. This concerns, for example, Westinghouse brand alloys (Zirlo, improved Zirlo, AXIOM), Framatome / Areva (alloys M5, Q) and Rosatom (alloys E110, E635). At the same time, in a number of zirconium alloys such components as tin, nickel and chromium were reduced or eliminated. Improved technology to minimize the content of hafnium in zirconium alloy.
- Comprehensive improvement of fuel assembly designs. During the development, some structural elements of the assemblies were excluded (covers and fuel assembly covers). There were solutions that increase the strength of the fuel assembly, its resistance to deformation, solutions that provide additional integrity of the fuel elements (introduction of anti-debris filters) and satisfy new regulatory requirements, for example, to seismic resistance. The design of fuel assemblies made collapsible, thereby allowing the replacement of individual fuel rods and continued operation.
Above are not all, but rather the most basic changes in the design of the fuel, which occurred since the fabrication of the first fuel assemblies.
Stumbling block
From the first point, one can guess that the current fuel assemblies for a long time of development have already managed to reach the maximum performance and safety indicators, but at least two factors now oblige designers to continue to improve nuclear fuel further.
Given the enormous power density of the active zone of a light-water reactor ~ 150 W / cm3, in combination with the possibility of introducing positive reactivity or loss of cooling in this complex system, the engineers who designed the reactors understood the importance of safety systems design from the very beginning.
In order to develop a mitigation strategy in the event of an accident, two types of events were taken as the basis for the design of safety systems: events based on the positive input of reactivity (reactivity insertion accident (RIA) ) and events based on loss of coolant (LOCA ). The main security systems were specially designed to respond to these project events.
But the experience of such accidents as at the Three Mile Island and Fukushima Daiichi NPPs proved that in case of multiple failures and overlaps of initiating events, active safety systems are not able to cope with the functions assigned to them, in particular the removal of residual heat from fuel assemblies in the active zone .
The heat transfer equation in its simple form explains well what happens in a nuclear reactor as an accident develops with a loss of heat dissipation: The

left side of the equation describes the temperature change ( T ) over time ( t); This change is also determined by the heat capacity of the materials in the core ( pcp ). In the general case, the first term on the right-hand side represents in a simplified form the heat exchange processes (conduction, convection, and radiation) for the removal of heat from the core. The second term is the amount of heat generated in the core ( Q ).
During the course of the aforementioned events, the cooling mode of the core is violated, the first component of the right side becomes numerically less and the heat Qgradually causes an increase in temperature. Since the reactor core becomes partially or completely bare (the water level drops, water is replaced by steam), the efficiency of heat removal from the core drops sharply, the fuel cell temperatures continue to rise, which is the beginning of the chemical and physical degradation of the fuel elements. The physical degradation of the fuel element shell begins at temperatures (700-1000 C) and causes swelling and rupture of the membranes.
Chemical degradation is mainly expressed by steam oxidation of zirconium. The key factor is the exothermicity of this reaction. And of course, the product of this reaction is explosive hydrogen. For example, ~ 125 kg of zirconium in each fuel assembly of the reactor under pressure produces about 820 MJ of heat and more than 2,700 moles of hydrogen gas when reacting with steam.
Depending on the design of the light-water reactor, about 25-40 tons of zirconium is present in the core, with full oxidation of which a huge amount of heat will be produced, in addition to the residual (at best) energy release of the fuel itself.

The magnitude of the thermal power of the system depending on the shutdown time of the reactor, taking into account the exothermic oxidation reaction of zirconium
Generated hydrogen, in turn, will not peacefully accumulate, and without proper operation of the systems for its disposal, will lead to a large-scale explosion or fire, while the active zone can penetrate the hull and take up the concrete of the reactor compartment.
The concept of fuel resistant to accidents
The apocalyptic scenario described above basically repeats the events at the Fukushima nuclear power plant in 2011. This event led to the revision of a number of nuclear safety standards, especially those related to serious design and beyond design basis accidents (with a complete blackout of the reactor facility and loss of coolant). Due to this accident, in many regions of the world, competition of nuclear power plants with other energy sources has intensified, which significantly increases the requirements for the economics of nuclear power plants, as well as their safety (with equal or sometimes losing economic indicators of projects, investors may prefer non-nuclear energy sources).
This factor significantly increases the requirements for all elements of the production of electricity in nuclear power plants, especially nuclear fuel. In this decade, efforts have been intensified to create a fundamentally new fuel that can withstand severe accident conditions while maintaining or improving economic performance and safety during normal operation. Many developments of this kind have received the collective name Accident Tolerant Fuel (ATF) - a fuel with increased resistance to accidents.
The philosophy of changes in the design of fuel assemblies is based on the substitution of materials for the main components of nuclear fuel, mainly TVEL shells and fuel pellets for materials that are more resistant to the processes occurring during the course of the accident.
TVEL shell
The main approach in the choice of fuel cladding materials for ATF fuels is the need to eliminate or reduce the degree of the vapor-zirconium reaction and, as a result, the generation of additional heat and hydrogen. A quick and obvious solution is to apply a protective coating to the surface of the zirconium shell. Thin coatings on the zirconium shell should have a minimal effect on the thermal and neutron-physical characteristics of the fuel. Scientists have found that chromium, aluminum, and silicon have good resistance to vapor oxidation at high temperatures. These impurities demonstrate stability in a high-temperature steam environment, despite the fact that they can react somewhat with steam.
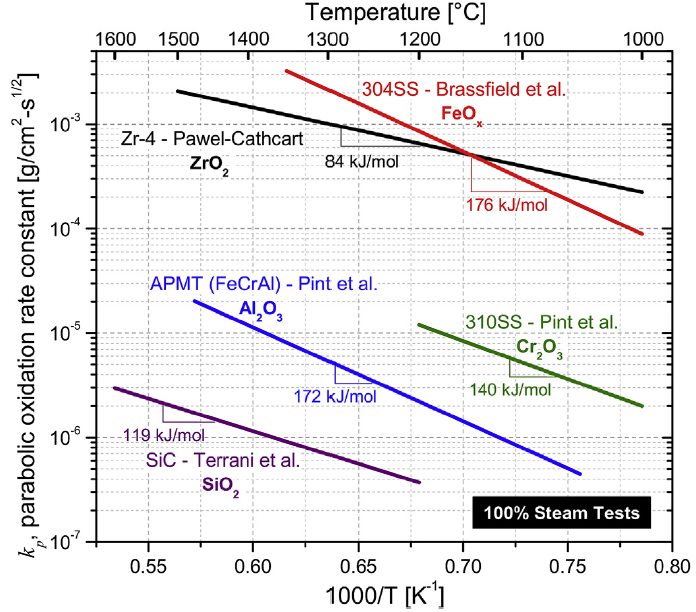
The rate of parabolic oxidation for various materials in a pair, depending on temperature
As can be seen from the graph, the oxidation rate of these materials, which form and, accordingly, are protected by their oxide films, is two orders of magnitude lower than the oxidation rate of zirconium. The reduction in steam oxidation rate directly affects the rate of heat and hydrogen evolution in the presence of heavy LOCA in the reactor core.
The coating of TVEL shells with metallic chromium is now considered the most promising technology for further development. Also considered to be promising shell TVEL without the use of zirconium, for example, materials FeCrAl, and SiC / SiC.

Testing of EnCore (Westinghouse) silicon envelope at temperatures above 1300ºC
Fuel cells
The second most important direction in the development of ATF fuel is the selection and justification of the material of the fuel matrix, which will have better thermal conductivity compared to classical ceramics. This, in turn, requires solving a number of problems: prevention of chemical reactions of the shell and fuel, swelling and damage to the shell of the fuel, localization of fission products, etc.
The Atomic Energy Institute of South Korea (KAERI) is working to create microelement tablets (microcell) for an increase in the retention capacity of fission products and better thermal conductivity compared to conventional uranium dioxide tablets.
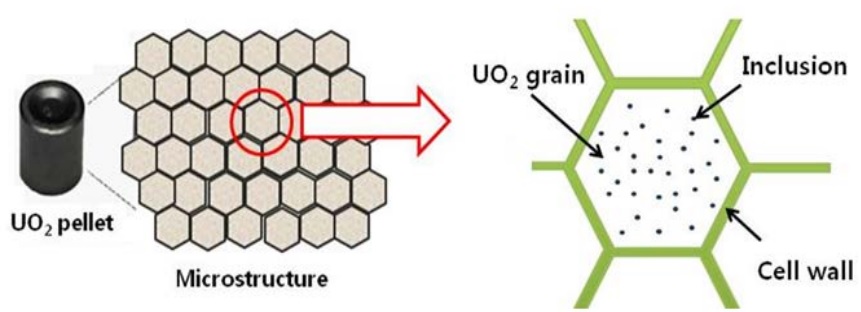
Micronutrient Fuel Tablet Concept
The illustration shows a conceptual illustration, where it can be seen that the grains or granules of UO2 are surrounded by a thin wall. The main task of creating such tablets is to reduce the yield of fission products from the tablet. Improved ability to retain fission products reduces stress corrosion cracking from the inside of a fuel cell caused by iodine and cesium.
This is expected to have a positive effect on the strength of the fuel rods. The microelement structure will also prevent massive tablet fragmentation in an accident, thereby providing additional retention of radioactive fission products.
The thermal conductivity of such tablets can be increased by adding materials with a high thermal conductivity, for example, using metals in the form of a wall of one element.
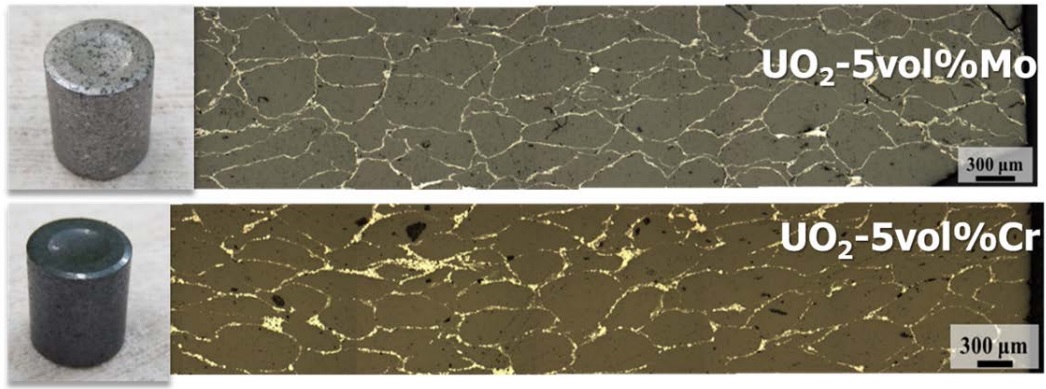
The typical structure of microelement tablets with metal.
This change will reduce the temperature in the center of the tablet under normal and emergency conditions of fuel elements operation.
To understand how the above innovations are implemented in practice, I will give the following example. Westinghouse creates tolerant fuel under the brand name EnCore, which is a tablet of uranium silicide U3Si2, enclosed initially (at the first stage of this program) in a shell made of chrome-plated zirconium alloy Zirlo.
As expected, the uranium silicide fuel will exceed the traditional dioxide by more than 5 times in thermal conductivity and ~ 1/5 in density, and the absorption of neutrons by a silicon carbide sheath should be ~ 1/4 less than in zirconium alloys.
Due to the last two parameters, the company intends to keep EnCore enrichment within 5%, which will facilitate its promotion in the market. In 2018, Westinghouse plans to set up pilot production of fuel elements in a chrome-plated zirconium sheath, in 2019, to begin their testing as part of regular fuel assemblies at Byron NPP in the USA.
Results
The changes described above are part of the actual projects being implemented now. Perhaps in the future we will witness the introduction of more exotic solutions.
So far, it is worth noting that the appearance on the market of cost-effective and new, in terms of safety, nuclear fuel projects will help strengthen the position of nuclear energy in the world.
Author: Mikhail Yatsenko.
Sources:
- Kurt A. Terrani "Accident tolerant fuel cladding development: Promise, status, and
challenges"; - IAEA-TECDOC-1797 "Accident Tolerant Fuel Concepts
for Light Water Reactors"; - Materials of the journal "Atomic Expert" № 3, May 2018.