Ask Ethan: maybe the missing antimatter is hidden inside black holes?
- Transfer

The artist’s vision of the Kepler-42 planetary system. We have every reason to believe that all of it consists of matter, and not of antimatter, but black holes may tell us a completely different story - we have no opportunity to establish what they consist of.
One of the greatest cosmic mysteries of the whole Universe is why there is so much more matter in it than antimatter. The laws of physics, as far as they are known to us, allow us to create and destroy matter and antimatter strictly in equal shares. However, looking at the stars, galaxies and large-scale structures of the Universe, we find that they are all made of matter, and the amount of antimatter is negligible. This cosmic mystery makes many people speculate that there may have been an equal amount of antimatter, and then it somehow separated from matter. Is it possible, and could antimatter hide in black holes? Our reader asks:
There is a mystery of the presence of matter and the absence of an appropriate amount of antimatter. Some remote and old black holes were formed much faster than current theories can predict. Can the missing antimatter hide inside these prehistoric black holes? Is the mass of supermassive black holes comparable, at least approximately, with the amount of missing antimatter?Thought exciting. Let's dive into this theory and see.

The galactic cluster of MACSJ0717.5 + 3745 should consist of matter, like us, or on the line of sight we would see evidence of the annihilation of matter with antimatter.
In whatever direction we observe the Universe, everywhere we see the same thing: galaxies and stars, in all directions and all places of space, at least on average. On small scales, of course, galaxies accumulate together, but if you look at the largest scales, the Universe will have on average identical properties everywhere (for example, density). If in some place there existed a galaxy consisting of antimatter, but not of matter, we would see a huge amount of evidence of the annihilation of matter / antimatter, and a shortage of matter on the border between matter and antimatter. The fact that we have nowhere found such evidence — neither in individual galaxies, nor in clusters of galaxies, nor in colliding clusters of galaxies — tells us that 99.999% + the Universe consists of matter, like us, and not of antimatter.

In clusters, galaxies, neighboring star systems, in our own solar system - everywhere there are serious restrictions on the proportion of antimatter presence. There is no doubt that matter dominates everywhere in the Universe.
And this is strange, because, according to the current understanding of the laws of physics, we are not aware of the mechanisms that would allow us to create more matter than antimatter. The symmetry of matter and antimatter, in terms of particle physics, is postulated even more strictly than you could imagine. For example:
- every time a quark occurs, an antiquark is created,
- every time a quark is destroyed, an antiquark is destroyed,
- every time when a lepton appears or is destroyed, the same antilepton of the same lepton family is created or destroyed,
- each time a quark or lepton interacts, collides or decays, the total number of quarks and leptons at the end of the reaction (quarks minus antiquarks, leptons minus antileptons) remains constant.
The only way to get more matter in the universe is to also create more antimatter.
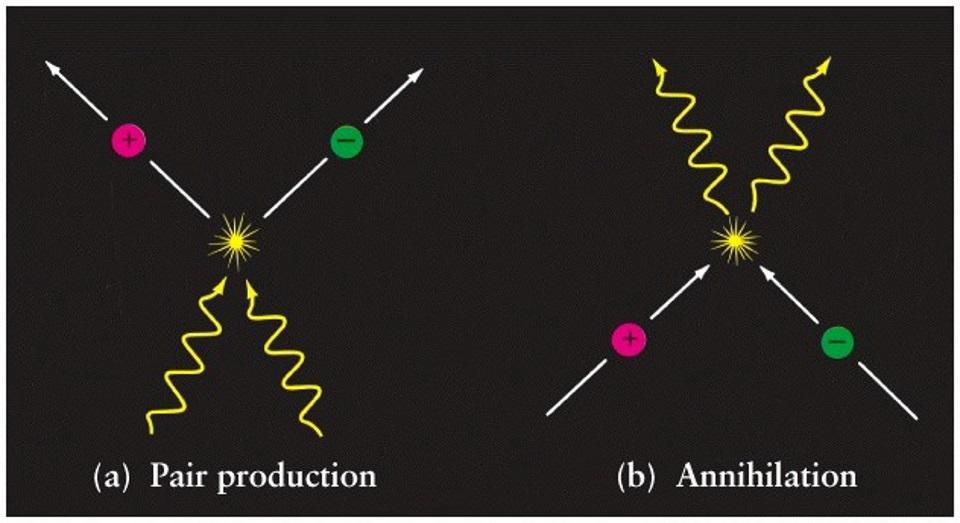
Getting a pair of matter / antimatter (left) from pure energy [photons] - the reaction is completely reversible (right), matter / antimatter can annihilate, turning into pure energy. The process of creation and annihilation, obeying the equation E = mc 2, is the only known way to create and destroy matter or antimatter.
The standard interpretation of these facts is that while it is not clear how, but in the past of the Universe there appeared more matter than antimatter. In the standard picture of the hot Big Bang, when the Universe was very young, a huge amount of particle-antiparticle pairs were created for all known (and even those that we will discover later) particles. This is because, at high temperatures and densities, it is possible to spontaneously obtain new particle-antiparticle pairs from pure energy, thanks to E = mc 2 Einstein. In equal quantities, these pairs annihilate, again producing pure energy (photons). With the cooling of the universe, the energy for making new pairs ends and annihilation begins to dominate.

With the expansion and cooling of the Universe, unstable particles and antiparticles decay, matter-antimatter vapors annihilate, and photons are no longer able to collide with energy large enough to create new particles.
If we didn’t have the asymmetry of matter and antimatter, then the Universe would turn out, in which for each proton there are 10 20photons and one antiproton. There would be about as many electrons and positrons as there are protons and antiprotons, and that’s all. Instead, however, we observe the Universe, in which for every proton there are “only” 1-2 billion photons. We assume that in the early Universe a certain asymmetrical process took place that gave rise to this asymmetry. A simple example would be the creation of a new set of particles and antiparticles with different preferences about the decay channels, which could lead to a slight advantage of matter over antimatter.

A symmetric collection of bosons of matter and antimatter (X, Y, anti-X and anti-Y) could, with certain properties of the Theory of Great Unification , generate the asymmetry of matter-antimatter that we observe in the Universe today.
What about our new idea? What if, at some of the early moments, something caused anti-matter to collapse into black holes, leaving normal matter behind? After all, we really see very early supermassive BHs in large quantities! However, their origin is not necessarily a problem or a good motivation to support such an unusual idea. Everything that can be explained without the involvement of new physics should be explained in the same way, and as for supermassive BHs, we think that this is all a matter of a direct collapse. Some BHs do not need to appear before a star, which would burn and turn into a supernova; they simply collapse, which could generate quite large “seeds” of BH quickly enough to explain the presence of young quasars that we are seeing today.

Remote massive quasars in their core have supermassive black holes. They are very difficult to create without having a large “seed”, but the black hole of direct collapse can quite elegantly solve this riddle. We can also calculate the masses of central BH based on the properties of quasars, and, although they are incredibly large, they contain much less mass than in the normal matter of the Universe.
So do not look in the direction of supermassive BH. There is another idea about primary black holes.which is periodically resurrected as a candidate for the explanation of dark matter. They cannot be too light, or they would evaporate very quickly; they cannot be too heavy, or we would notice them. Most of the mass intervals in which the mass of primary BHs should be placed, claiming to explain the missing mass of the Universe, have already been excluded or taken in a very rigid framework. To create a primary BH, a density fluctuation (deviation from the average density) of 68% is necessary, but in the early Universe the largest fluctuations did not deviate from the average density by more than 0.006%. In fact, the only permitted interval of masses, which the primary BHs could fit in for a fairly large proportion of dark matter, was already rejected by LIGO. His observations on the speed of the BH merger suggest that

Restrictions on dark matter, consisting of primary BH. The only available “window” in which dark matter can consist of black holes has recently been closed by restrictions obtained from LIGO on the stochastic background of black holes of just such an interval of masses.
Further, we were able to estimate the total BH mass in the Universe, and it is about 0.007% of the total energy . Considering that normal matter is 700 times more than BH, antimatter cannot hide in them; antimatter did not generate BH.
But we have another way to find out: the laws of physics have symmetry rules that must be satisfied by matter and antimatter. One of these rules applies to forces acting on particles: whatever force acts on a particle, a force of the same magnitude must act on an antiparticle (possibly with the opposite sign). But it works in both directions, so there can be no forces acting only on antimatter. If you want something to affect the antimatter of the Universe, it should also affect matter.

The replacement of a particle by an antiparticle and their simultaneous reflection in a mirror is CP-invariance. If the anti-mirror decays not as normal, the invariance is broken. The symmetry in time, or T, is broken if the CP is broken. The combined symmetry of C, P and T should be maintained according to the current laws of physics, which affects which interactions can occur and which cannot.
Therefore, taking into account the existing laws of physics, we are sure that antimatter could not have collapsed and turned into BH, leaving normal matter behind. If the amount of dark and normal matter would be the same, such reasoning would make sense, but the following points:
- we do not need exotic physics for the appearance of supermassive BH in the early Universe;
- the primary BHs do not fit into the idea of the formation of the structures of the Universe, and the existence of a large number of them, for the most part, is excluded;
- antimatter is forbidden to experience interactions leading to their transformation into BH, despite the fact that BH could not be made from normal matter.
enough to return to the standard picture. Somehow, the Universe produced more matter than antimatter, at some point in a very distant past, and that is why we were able to appear. How exactly this happened is one of the greatest unsolved mysteries of today's physics.
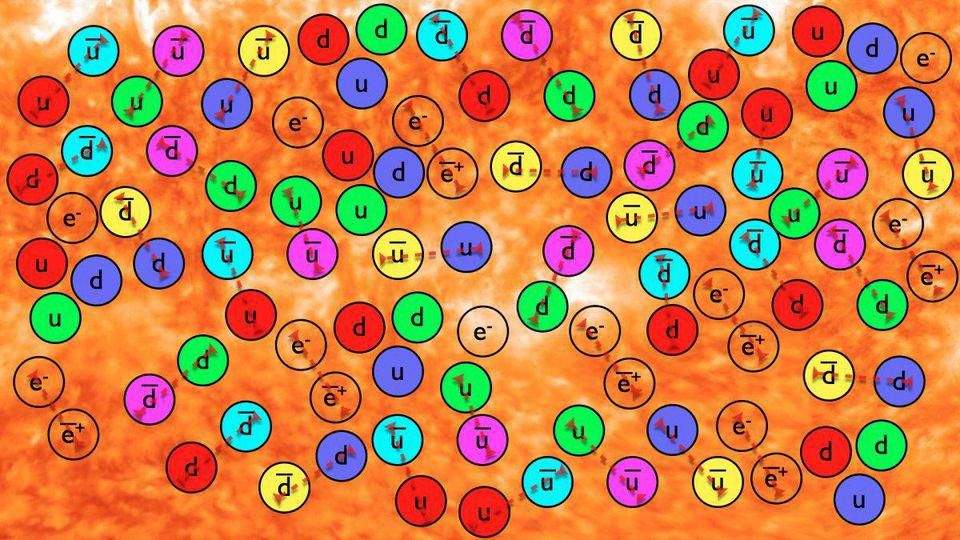
The early Universe was filled with matter and antimatter that were in a sea of radiation. But when all this after cooling annihilated, a little bit of matter remained. The exact description of this process is known as the problem of baryogenesis , and remains one of the greatest unsolved problems in physics.