Ask Ethan # 76: A Very Early Universe
- Transfer
What happened when everything was so hot that matter and antimatter formed spontaneously?
From the special theory of relativity it follows that mass and energy are different manifestations of the same thing - a concept unknown to the average mind.
- Albert Einstein
Every week you have the opportunity to send questions and requests to become the star of our daily column. This week we are being transferred to the earliest stages of the Big Bang thanks to Wayne King, who asks:
There is a period about which we know little, it is the period of the annihilation of particles and antiparticles. Was this “matter” in the sense of protons and positrons? And what happened to the neutrons? Or was it some form of compressed energy from the field of quantum chromodynamics? How did she appear? Did anything remain in the annihilation process? How much energy was released, and where did it go?
Most authors are simply limited to general replies, describing this topic.
What is Wayne talking about? Let's start with the current state of the universe, and press the "rewind" back.
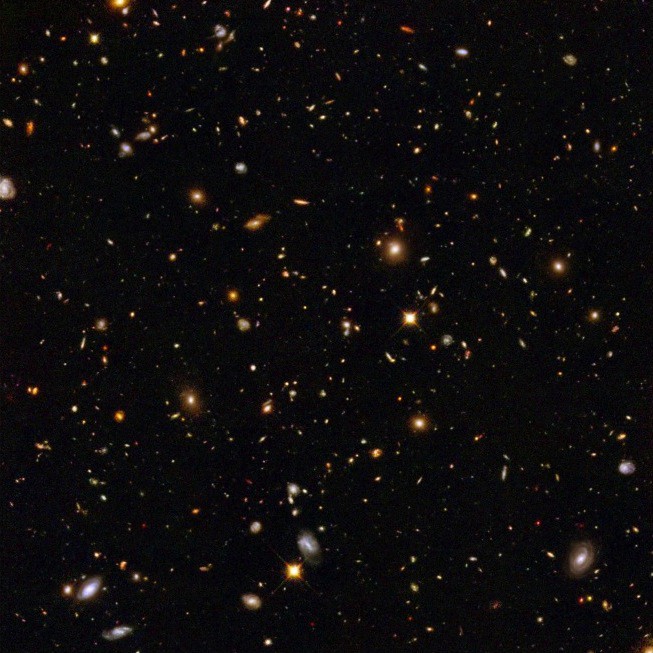
Today’s Universe is filled with stars bound into giant galactic structures, and on a large scale into groups, clusters and intersecting filaments. In the part that we can observe, there are at least hundreds of billions of galaxies scattered over distances of tens of billions of light years.
But how did the universe become like this? It expanded from a denser, more compact, uniform, and hot state. Now everything is so far scattered, since the Universe has been expanding for a very long time.
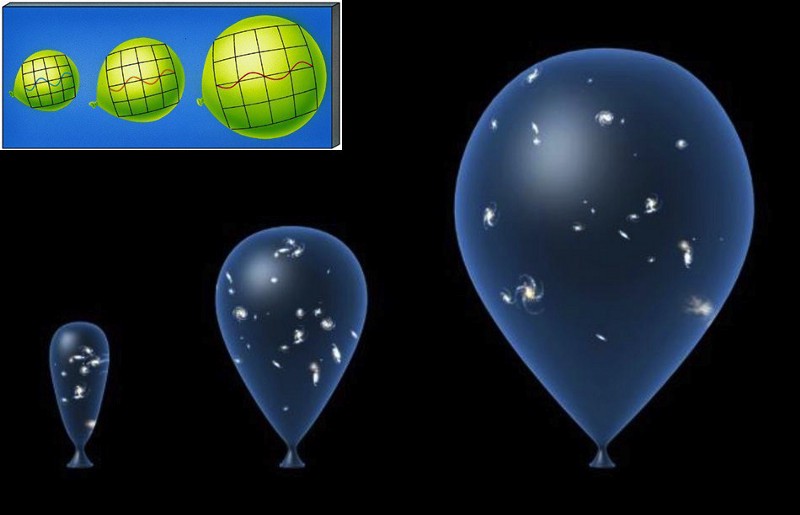
Extrapolating back, we find that a parameter that is not very important today - the temperature of the Universe, which is only 2.7 K - affects the Universe more and more. With low density and energy, these remaining photons do not affect anything, they are only displayed as “snow” on the third channel, if you are still using analog TV with antenna antennae.

But when the Universe was younger and smaller, these photons were not only more dense, since the volume of the Universe was smaller, but also hotter, since the wavelength of the photon determines its energy. Extrapolating back, we will see how microwave radiation turns into infrared, the temperature rises from a few degrees above absolute zero to two-digit numbers, three-digit, then exceeds room temperature, the boiling point of water, and begins to compete with the temperature of a burning star. At some point, everything becomes so hot that even neutral atoms cannot form, since the electrons that form them are knocked out of the orbits by a sea of photons.

If we rewind some more, we will arrive at the moment when atomic nuclei cannot form, since they are broken into separate protons and neutrons. And, as a result, we can go so far in time when the Universe was only one second old, and the photons were so energetic that matter and antimatter could spontaneously appear in equal quantities. Before the Universe expanded and cooled down after going through this phase, it represented only a “soup” of matter, antimatter and radiation, in which spontaneous annihilation of matter and antimatter into pure energy was balanced by the spontaneous appearance of particles of matter and antimatter from pure energy . The most famous Einstein equation, E = mc 2 , works in both directions.

The higher your energy, the more heavy pairs of particles you can spontaneously create. Having reeled back far enough when the average energy in the Universe was large enough to create pairs of true-anti-genuine quarks (the heaviest known particle), we will see that then the photons were much smaller than they are now.
From what?
This is because a particle-antiparticle pair can annihilate with the formation of two photons, and at sufficiently high energies the photons can interact with the appearance of a particle-antiparticle pair!

And although today there are a certain number of photons, imagine all the fundamental particles from the Standard Model, both massive and massless. All six quarks and antiquarks, three colors each, three charged leptons, three neutrinos, along with their antiparticles, eight gluons, three weak bosons, a photon and a Higgs boson, with all the allowed spin configurations.
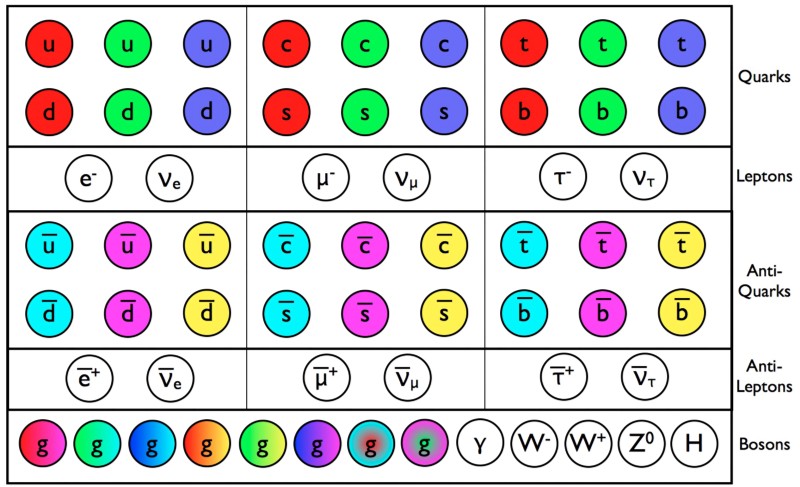
Instead of just photons, this energy is distributed equally among all kinds of particles. (In accordance with the Maxwell-Boltzmann energy distribution and the corresponding statistics: Fermi-Dirac for fermions and Bose-Einstein for bosons). When energy and temperature are high enough, particle / antiparticle annihilation occurs all the time, but with the same frequency as particle / antiparticle production.
As the universe expands and cools, the frequency of annihilation decreases as it becomes harder for particles to find their antiparticles, but the creation frequency drops even more — the energy drops below the threshold required for creation, as a result the frequency of creating pairs decreases exponentially.

Fortunately, almost everything is unstable, so here’s what happens as the Universe expands and cools (in order) from the “sea” state, in which everything (particles and antiparticles of all kinds) floats enough:
- The pairs of true-anti-genuine quarks cease to appear, and the remaining ones annihilate or decay.
- A pair of Higgs bosons cease to appear, and the remaining annihilate or decay. This roughly coincides with the violation of electro-weak symmetry.
- Z_0 cease to appear spontaneously, and most of the rest break up.
- W + / W- pairs cease to appear, and most of the remaining ones break up.
- The lower / anti-lowering, tau / antitau pairs, enchanted / anti-truncated quarks cease to appear, and the remaining annihilate and / or decay.
In all cases, the annihilation or decay of more massive particles leads to the heating of all remaining ones.
Then something interesting happens: before the Universe cools down to the next threshold to stop the production of strange / anti-strange quarks, it is made sparse and cold enough to go from quark-gluon plasma to individual baryons (combinations of three quarks), antibaryons (combinations of three antiquarks), and mesons (combinations of quarks and antiquarks). And here confinement happens for the first time.
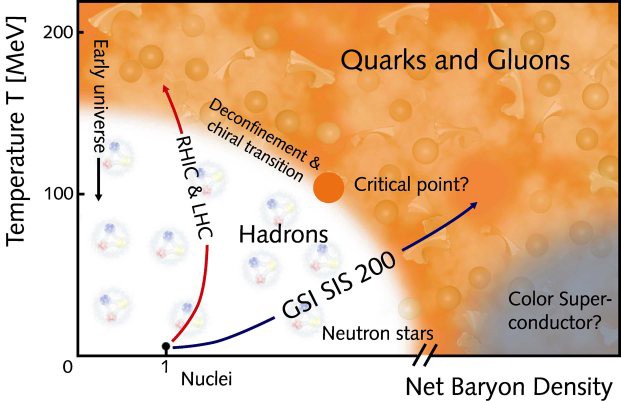
After this, the following annihilations and decays occur:
- all particles containing strange / antistran quarks decay / annihilate;
- all unstable baryons, antibaryons, and mesons decay / annihilate (except neutron, antineutron, and charged pions);
- annihilate neutrons / antineutrons and protons / antiprotons, leaving behind a few protons and neutrons representing the asymmetry of matter / antimatter;
- the creation of charged pions stops, and they annihilate / decay;
- the creation of muons / anti-muons stops, and they annihilate / decay.
At this moment, only a small number of protons and neutrons, a large number of pairs of electrons and positrons, neutrinos / antineutrinos and photons remain in the universe. Yes, and still dark matter, from whatever it was (and it was always present), which, according to our assumptions, does not interact with other particles.
You may decide that further electron / positron annihilation occurs, but first two other events occur.
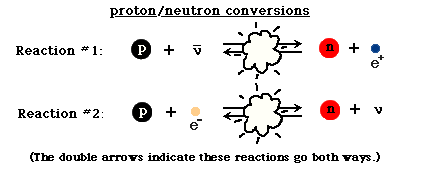
First, protons and neutrons play a game: protons try to combine with electrons to form neutrons and neutrinos, and neutrons and neutrinos try to go the other way, producing protons and electrons. Also, protons and antineutrinos can be combined and create neutrons and positrons, as well as the reverse reaction is possible. Within a few milliseconds, which is a decent amount of time for our history, these reactions occur with the same frequency. But with a decrease in energy and temperature, a small mass difference between the proton and the neutron begins to affect, and reactions with the creation of protons from neutrons become a little easier to go than reactions with the creation of neutrons from protons. By the time the age of the Universe approaches the second, the ratio of protons and neutrons changes in it from 50/50 to 85/15 in favor of protons.

Then weak interactions — interactions that allow neutrinos to exchange energy with other types of particles, and allowing transitions between photons and neutrons to take place, are frozen. This means that the frequency of interactions, the energy and the effective cross section become too small for neutrino and antineutrino to take part in reactions occurring in space. Up to this point, electrons / positrons, neutrinos / antineutrinos, and photons all received a proportional fraction of the energy from the annihilation. But when neutrinos and antineutrinos freeze, they stop participating in this game.

And so, when the final phase of annihilation happens, when the Universe cools so much that electron / positron pairs are no longer created, and they simply annihilate, leaving enough electrons to compensate for the electrical charge of the protons), they pour all the energy into photons, not neutrinos and antineutrino.
Therefore, the temperature of the microwave cosmic background radiation — the background of the photons remaining after the Big Bang — is measured at 2.725 K, and the background temperature of the remaining neutrinos should be around 1.95 K or, more precisely, (4/11) 1/3 of the temperature of the photons.
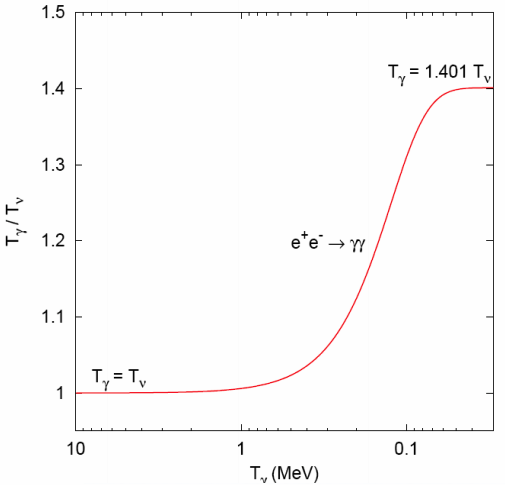
And also because of this - after three minutes with a trifle - some of the remaining neutrinos disintegrated, raising the ratio of protons to neutrons to ~ 87.6 / 12.4. At this step, the photons were finally cooled enough so that the formation of the first elements heavier than hydrogen could begin - the Big Bang nucleosynthesis. Therefore, it was precisely this correlation of hydrogen and helium after the Big Bang that happened to us: because of the roles played by all these particles in the early stages of the Universe.
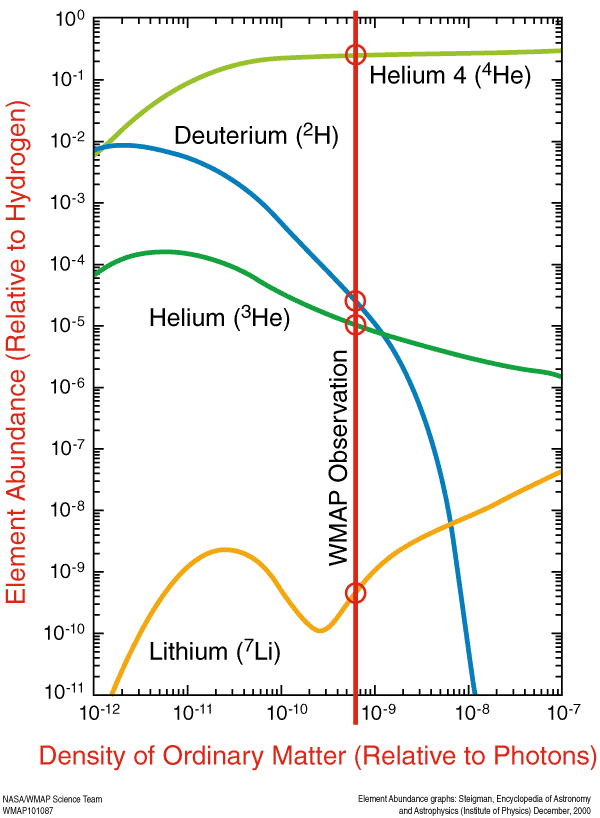
Someday I hope to inform you about the detection of the cosmic background neutrinos. Last month, his discovery was announced at an AAS meeting, but no work on this topic has yet appeared. I think I brought as much information as possible so as not to turn you into theoretical physicists, and I hope that the article was well balanced to meet your needs. So far this is the best of the stories of the appearance of particles in the Universe and their behavior in the early stages of the hot Big Bang, and then during cooling, annihilation and decay.
Thank you for the wonderful question, and I hope that the explanation has been made clear for you and for the rest. Send me your questions and suggestions for the following articles.