
Frozen time. Scientific Approaches to Dating
- Tutorial
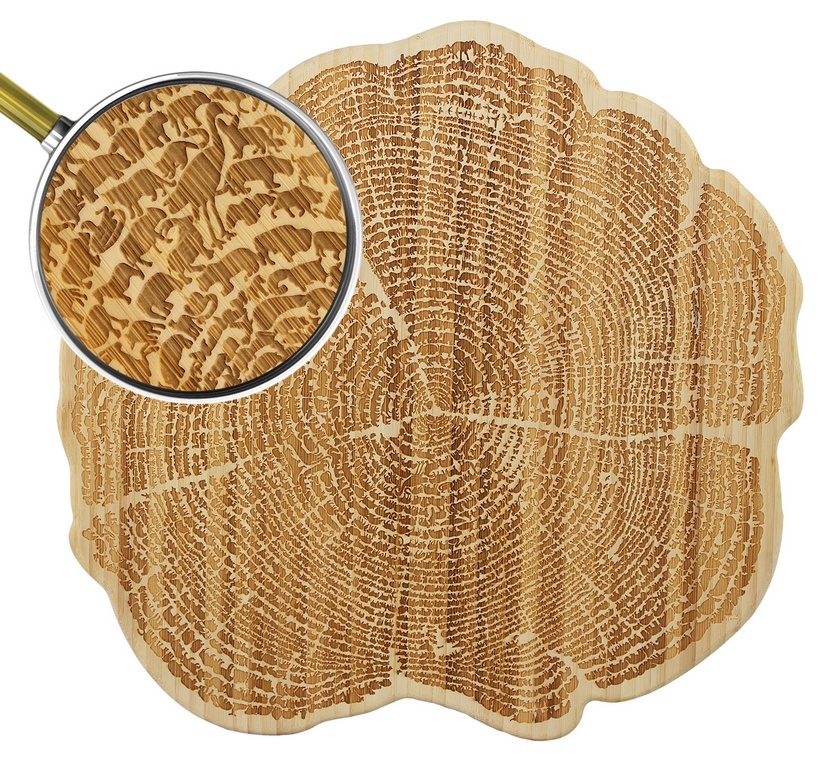
Today's story will be about the methodology of science, in particular about how we can determine the age of archaeological finds, what basic methods are used and what physical principles and processes underlie them.
The beauty of scientific dating methods is that they are complementary and mutually verifiable, that is, with the help of one method we can check the correctness of another and vice versa, making amendments to it if necessary. Also, these “clocks” cover a huge time range - about 9 orders of magnitude (actually more, but for historical purposes, “fast” clocks are useless, the scale of evolutionary time covers seven or eight orders of magnitude).
This can be compared with the work of late criminologists, where there are no direct witnesses from the "crime scene" and who have found only his tracks.
There are many things in science that are inaccessible to direct observation. This is one of the reasons for distrust and resistance to science at the “everyday” level. Today, despite the ever-widening gap in knowledge between “scientists” and “ordinary people,” significant efforts must be made so that people do not have the impression a la“These scientists themselves cannot explain anything, because they use the data of the same scientists who took this data from the ceiling.” Unfortunately, this is precisely the opinion that exists outside the scope of science, in particular, among the many “refutants” of history — somewhere in the kitchen or in the garage. Of course, doubts in science are useful, because any theory that claims to be scientific must be fundamentally falsified. The trouble is that in order to cast doubt on the methods described below, it is necessary to falsify facts from biology, physics, geology, archeology, history and chemistry.
All watches can be conditionally divided into two categories - counting (for example, oscillations of a pendulum or a quartz crystal in a household clock) - or measuring (for example, the time flow of any non-cyclic processes). And those and other hours at some (fortunately, necessary for us) moments can either “reset” or stop, fixing events. Let's start with the fastest hours.
Dendrochronology.

On the scale we need, for example, historical, the counting clocks used in dendrochronology are very convenient — these are annual rings of trees. For example, they can be used to determine in which year a tree was cut down that was used to build a house or build a cult several centuries ago (in fact, there is a continuous dendrochronological scale of about 11,500 years).
How does this method work? Many people know that to determine the age of a recently felled tree, you need to count the rings in its trunk, considering the present ring as the outer ring. The rings reflect changes in the growth rate in different seasons of the year - in summer or winter, in the dry season and in the rainy season, and are especially pronounced in high latitudes, where there is a strong difference between the seasons. At the same time, to determine the age, it is not necessary to cut down a tree. You can drill a hole to the middle of the tree and remove the sample. But a simple ring count will not show in which century the log from your house or the mast of your ship was alive. If you need to date long dead wood, you have to look at the characteristic pattern of the rings. Just as the presence of rings means annual cycles, so some years are worse than others, because the weather changes every year: drought will slow the growth rate, and a rainy year will accelerate it; there are cold and warm years, and even the years of El Nino or the eruptions of Krakatoa. Years with poor climatic conditions for wood produce narrower rings than good ones. And the pattern of narrow and wide rings in a particular region, created by a specific sequence of different years, is a characteristic “imprint” that accurately marks the years of formation of these rings, recognizable from tree to tree. In addition, you can always take a sample of the material from the desired ring for radiocarbon dating (more on this below). created by a specific sequence of different years, is a characteristic “imprint” that accurately marks the years of formation of these rings, recognizable from tree to tree. In addition, you can always take a sample of the material from the desired ring for radiocarbon dating (more on this below). created by a specific sequence of different years, is a characteristic “imprint” that accurately marks the years of formation of these rings, recognizable from tree to tree. In addition, you can always take a sample of the material from the desired ring for radiocarbon dating (more on this below).
All this, of course, is good, but the rare of the living trees were alive in the time of Peter the Great, not to mention the Bronze Age or earlier. There are trees that live for millennia, but most of them are cut down when they are not even a hundred years old. How is a reference collection of rings created for more ancient times? I think you already guessed.

Overlapping. The rope may be a hundred meters long, but the individual fibers in it are much shorter. To use the overlap principle, you take reference patterns of patterns whose date can be set on modern trees.
Then you look for a pattern of old rings of modern trees and determine the correspondence of the pattern among the younger rings of long dead trees. Then you identify the pattern of the old rings of these long dead trees, and look for the same pattern in the young rings of even older trees, etc. In practice, this method is used only in archaeological periods, on the scale of several thousand years.
By the way, this is not the only system that promises accuracy up to a year. Sedimentary layers are deposited in glacial lakes. Like annual rings, they vary seasonally and theoretically, the same principle can be used here, with the same degree of accuracy. Corals also have growth rings, as do trees. They were used to determine the dates of ancient earthquakes. Most other dating systems available to us, including all radioisotope methods, are accurate only within the margin of error, which is proportional to the scale of the measured time.
Radioisotopes.
For those who have successfully forgotten physics, first I will tell you basic information about the structure of matter and what radioisotopes are, because here we are dealing with physical processes.
All matter consists of elements that chemically interact with other elements. In nature, there are 92 elements minus technetium, a little more if we count artificially synthesized elements. The atomic theory of the structure of matter, which I think even creationists accept, tells us that elements consist of characteristic atoms, which are the smallest particles into which an element can be divided so that it does not cease to be this element. What does an atom like nitrogen or copper or carbon look like?

The atom consists of three types of particles, at least in the Bohr model. We are already familiar with electrons. Two other particles, much larger, are called protons and neutrons, and they are in the nucleus and their size is almost the same. The number of protons is constant for any particular element and is equal to the number of electrons. This number is called the atomic number and is written in the lower index near the name of the element. This is a unique characteristic of the element, and there are no gaps in the list of atomic numbers of the famous periodic system [Mendeleev]. Each number in it corresponds to exactly one and only one element. The atomic number 1 is hydrogen, 2 is helium, 3 is lithium, and so on, up to 92 for uranium.
Protons and electrons carry an electric charge of the opposite sign. We call one of them positive and the other negative, in accordance with an arbitrary agreement. These charges are important in the formation of chemical bonds of elements with each other, mainly through the interaction of electrons. Neutrons in an atom are connected in the nucleus with protons and have no charge, and they do not participate in chemical reactions. Neutrons, protons and electrons in any element are exactly the same as in any other. There is no such thing as an oxygen proton, or a potassium electron, or a copper neutron. Proton - it is everywhere a proton, but what makes a copper atom copper is that it has exactly 29 protons (and 29 electrons). What we think in everyday terms as copper is a matter of chemistry. Chemistry is the dance of electrons. Its whole essence lies in the interaction of atoms through their electrons. Chemical bonds are easily destroyed and re-created, because only electrons are separated or exchanged in chemical reactions. The forces of attraction inside atomic nuclei are much more powerful. That is why “atom fission” sounds so ominous, but it can occur in “nuclear” (as opposed to chemical) reactions, and the radioactive clock is based on them.
Electrons have a negligible mass, so the total mass of an atom, its “atomic mass", is equal to the total number of protons and neutrons. As a rule, it is slightly more than double the atomic number, because usually in the nucleus, as a rule, there are more neutrons than protons. The atomic mass is written by the superscript next to the element designation in the periodic table. Unlike the number of protons, the number of neutrons in an atom is not a unique feature of an element. The atoms of any particular element can be in different "versions" called isotopes, differing in the number of neutrons, but always with the same number of protons. Some elements, such as fluorine, have only one naturally occurring isotope. The atomic number of fluorine is 9, and its atomic mass is 19, from which it is clear that it has 9 protons and 10 neutrons. Other elements have several isotopes. Lead has five common isotopes. They have the same number of protons (and electrons) - 82, which is the atomic number of lead, but with different atomic masses - from 202 to 208. Carbon has three isotopes found in nature. Carbon-12 is ordinary carbon with the same number of neutrons and protons - 6. There is also carbon -13, which is too short-lived for our purposes, and carbon -14, which is rare, but not enough to be useful for dating organic samples.
The next important theoretical fact is that not all isotopes are stable. Lead-202 is an unstable isotope, and lead-204, -206, -207 and -208 are stable. “Unstable” means that atoms spontaneously decay into something else, at a predictable speed, albeit at unpredictable times. Decay rate predictability is the key to all radiometric watches. A synonym for the word "unstable" is "radioactive." There are several types of radioactive decay, suitable as clocks in which neutrons participate. In one form (β --decay) a neutron turns into a proton. This means that the atomic mass remains the same (the protons and neutrons have the same mass), and the atomic number increases by one, so the atom becomes a different element, one cell to the right in the periodic system. For example, cesium-55 turns into barium-56. In another form of radioactive decay (β + decay), on the contrary, the proton turns into a neutron. The atomic mass remains the same again, but this time the atomic number decreases by one, and the atom becomes the next element to the left of the periodic system. The third type of radioactive decay ( electron capture) has the same result. A proton is able to capture one of the electrons in the shell of its atom and turn into a neutron (emitting a neutrino). Again, there are no changes in the atomic mass, the atomic number decreases by one, and the atom turns into the next element to the left of the periodic system. There is also a more complex type of decay, in whichan atom emits a so-called alpha particle. It consists of two neutrons and two protons “glued” together (or the nucleus of a helium atom without electrons). This means that the atomic mass decreases by four, and the atomic number decreases by two. An atom turns into that element, which is two cells to the left of the periodic table. An example of alpha decay is the conversion of a very radioactive isotope of uranium-238 (with 92 protons and 146 neutrons) into thorium-234 (with 90 protons and 144 neutrons).
Now to the point. Each unstable isotope decays at a precisely known rate, for each isotope of its own. In all cases, the decay is exponential. The generally accepted measure of decay rate is the “half-life”. This is the time taken to decay half of its atoms. The half-life is the same and does not depend on how many atoms have already decayed. For example, the half-life (T½) of carbon-14 is 5730 ± 40 years. In 2010, the maximum age of the sample, which can be precisely determined by the radiocarbon method, is about 60,000 years, that is, about 10 half-lives of 14 C. During this time, the content of 14 C decreases by about 1000 times (about 1 decay per hour per gram of carbon) and we will have to turn to a slower clock.
Potassium Argon Method
The isotope often used on an evolutionary time scale is potassium-40 with a half-life of 1.26 billion years, and it will be used as an example to explain the whole idea of a radioactive clock. This "clock" is called potassium-argon, since argon-40 (it is per cell to the left of the periodic system) is one of the elements into which potassium-40 decays (the other, as a result of another type of radioactive decay, is calcium-40, located on unit to the right of the periodic table). If you start with a certain amount of potassium-40, then after 1260 million years, half of the potassium-40 will decay into argon-40. This is called the half-life. After another 1.26 billion years, half of what remains (1/4 of the original) and so on will decay. Over a period of time shorter than 1.26 billion years, a smaller amount of starting potassium will decompose accordingly. Suppose we have some potassium-40 in a closed system, without argon-40. After several hundred million years have passed, the scientist comes across this confined space and measures the relative proportions of potassium-40 and argon-40. From this fraction, regardless of the absolute quantities, knowing the half-life of potassium-40 and assuming that there was no argon at first, we can estimate the time elapsed since the start of the process, that is, from the time that the clock was “reset”. Note that we must know the ratio of the parent (potassium-40) and daughter (argon-40) isotopes. Moreover, as mentioned earlier, it is necessary that our watch be reset to zero. After several hundred million years have passed, the scientist comes across this confined space and measures the relative proportions of potassium-40 and argon-40. From this fraction, regardless of the absolute quantities, knowing the half-life of potassium-40 and assuming that there was no argon at first, we can estimate the time elapsed since the start of the process, that is, from the time that the clock was “reset”. Note that we must know the ratio of the parent (potassium-40) and daughter (argon-40) isotopes. Moreover, as mentioned earlier, it is necessary that our watch be reset to zero. After several hundred million years have passed, the scientist comes across this confined space and measures the relative proportions of potassium-40 and argon-40. From this fraction, regardless of the absolute quantities, knowing the half-life of potassium-40 and assuming that there was no argon at first, we can estimate the time elapsed since the start of the process, that is, from the time that the clock was “reset”. Note that we must know the ratio of the parent (potassium-40) and daughter (argon-40) isotopes. Moreover, as mentioned earlier, it is necessary that our watch be reset to zero. you can estimate the time elapsed since the start of the process, that is, from the time that the clock was “reset to zero”. Note that we must know the ratio of the parent (potassium-40) and daughter (argon-40) isotopes. Moreover, as mentioned earlier, it is necessary that our watch be reset to zero. you can estimate the time elapsed since the start of the process, that is, from the time that the clock was “reset to zero”. Note that we must know the ratio of the parent (potassium-40) and daughter (argon-40) isotopes. Moreover, as mentioned earlier, it is necessary that our watch be reset to zero.
But what is meant by "zeroing"? Crystallization process.
Like all radioactive clocks used by geologists, potassium-argon time counting only works for so-called igneous rocks. Igneous rocks solidify from molten rocks - underground magma in the case of granite, lava from volcanoes in the case of basalt. When a rock solidifies, it crystallizes and forms granite or basalt. These, as a rule, small, transparent crystals, like quartz, are too small to look like crystals for the naked eye. Some of them, such as feldspars and mica, contain potassium atoms. Among them are atoms of the radioactive isotope potassium-40. When a crystal forms at the time of magma solidification (the system “ closes ””), Potassium-40 is present, but there is no argon (it is assumed that the bubbles of this gas, if any, rose to the surface of liquid lava and mixed with atmospheric air). The clock is "zeroed" in the sense that there are no argon atoms in the crystal. After millions of years, potassium-40 decays slowly, and, one after another, the argon-40 atoms replace the potassium-40 atoms in the crystal and remain in it like in a trap. The cumulative amount of argon-40 is a measure of the time elapsed since crystallization. But this value makes sense only when expressed as the ratio of potassium-40 to argon-40. When the watch was reset, the ratio was 100% in favor of potassium-40. In 1.26 billion years, the ratio will be 50 to 50. After another 1260 million years, half of the remaining potassium-40 will turn into argon-40, and so on. Intermediate proportions show intermediate times from when the crystal clock was reset. Thus, by measuring the ratio40 K / 40 Ar in a piece of igneous rock today, it can be said when the rock crystallized. Igneous rocks, as a rule, contain many different isotopes, and not only potassium-40. The positive point is that igneous rocks in this piece harden at the same time, resetting all hours, which is very convenient for dating. However, during the crystallization of the mineral, argon capture from the outside can occur. How to distinguish this argon from what formed later during the decay of the 40 K isotope ? We can proceed from the assumption that the captured argon had the same 40 Ar / 36 Ar isotope ratio as in the modern atmosphere. Measuring the amount of 36Ar, you can then calculate the amount of “pure” radiogenic argon 40 Ar.
However, there is a problem. Fossils are extremely rare in igneous rock. They form in sedimentary rocks such as limestone and sandstone, which are not solidified lava. They are found in layers of mud, silt or sand, gradually deposited on the bottom of the sea, lake or river. Sand or silt condenses over many centuries and hardens like stone. Remains that have fallen into sedimentary rock have a chance to fossilize (to survive as a fossil). Although only a small part of the corpses becomes fossil, sedimentary rocks are the only ones that contain fossils that are worth talking about.
Unfortunately, these rocks cannot be dated using radioactivity. It is likely that the individual particles of silt or sand that are part of sedimentary rocks contain 40 K and other radioactive isotopes, but, unfortunately, this watch is useless because it is not properly reset or reset at different times. Each grain of sand has a clock zeroed out at one time, probably long before the formation of these rocks and the burial of minerals that we are trying to date. So, in terms of timing, sedimentary rock is a continuous mess. The best we can do, and this is a pretty good “best”, is to use the age of volcanic rocks that are near or embedded in sedimentary rocks.
Dating a fossil literally does not require finding it pressed between two plates of igneous rocks, although this is a great way to illustrate the principle. In fact, a more sophisticated method is used. Recognizable layers of sedimentary rocks are found all over the world. Long before radioactive dating was discovered, these layers were identified and named: Cambrian, Ordovician, Devonian, Jurassic, Cretaceous, Eocene, Oligocene, Miocene. Devonian deposits are recognizable as Devonian, not only in Devon (the county in the southwest of England, which gave them their name), but also in other regions. They are clearly similar to each other, and they contain the same types of fossils. Geologists have long been aware of the order in which these deposits were deposited. Before the advent of the radioactive clock, we simply did not know when they formed. We could arrange them in order, because, obviously, the older deposits tend to lie below the younger deposits. The Devonian deposits, for example, are older than the deposits of the Carboniferous period (so named because coal is often found in this layer), and we know this because in those parts of the world where these two layers meet in one place, the Devonian layer lies under the Carboniferous (exceptions occur in places where we can say, on the basis of other evidence, that the rocks were inclined, or even turned upside down). It rarely happens that a complete set of layers is found - from the Cambrian in its lower part to the modern ones at the very top. But since the layers are so recognizable, their relative ages can be determined by building one after another and assembling them like a puzzle around the world. obviously, older sediments tend to lie below younger sediments. The Devonian deposits, for example, are older than the deposits of the Carboniferous period (so named because coal is often found in this layer), and we know this because in those parts of the world where these two layers meet in one place, the Devonian layer lies under the Carboniferous (exceptions occur in places where we can say, on the basis of other evidence, that the rocks were inclined, or even turned upside down). It rarely happens that a complete set of layers is found - from the Cambrian in its lower part to the modern ones at the very top. But since the layers are so recognizable, their relative ages can be determined by building one after another and assembling them like a puzzle around the world. obviously, older sediments tend to lie below younger sediments. The Devonian deposits, for example, are older than the deposits of the Carboniferous period (so named because coal is often found in this layer), and we know this because in those parts of the world where these two layers meet in one place, the Devonian layer lies under the Carboniferous (exceptions occur in places where we can say, on the basis of other evidence, that the rocks were inclined, or even turned upside down). It rarely happens that a complete set of layers is found - from the Cambrian in its lower part to the modern ones at the very top. But since the layers are so recognizable, their relative ages can be determined by building one after another and assembling them like a puzzle around the world. The Devonian deposits, for example, are older than the deposits of the Carboniferous period (so named because coal is often found in this layer), and we know this because in those parts of the world where these two layers meet in one place, the Devonian layer lies under the Carboniferous (exceptions occur in places where we can say, on the basis of other evidence, that the rocks were inclined, or even turned upside down). It rarely happens that a complete set of layers is found - from the Cambrian in its lower part to the modern ones at the very top. But since the layers are so recognizable, their relative ages can be determined by building one after another and assembling them like a puzzle around the world. The Devonian deposits, for example, are older than the deposits of the Carboniferous period (so named because coal is often found in this layer), and we know this because in those parts of the world where these two layers meet in one place, the Devonian layer lies under the Carboniferous (exceptions occur in places where we can say, on the basis of other evidence, that the rocks were inclined, or even turned upside down). It rarely happens that a complete set of layers is found - from the Cambrian in its lower part to the modern ones at the very top. But since the layers are so recognizable, their relative ages can be determined by building one after another and assembling them like a puzzle around the world. because in those parts of the world where these two layers meet in one place, the Devonian layer lies under the Carboniferous (exceptions are found in places where we can say, based on other evidence, that the rocks were tilted, or even turned upside down). It rarely happens that a complete set of layers is found - from the Cambrian in its lower part to the modern ones at the very top. But since the layers are so recognizable, their relative ages can be determined by building one after another and assembling them like a puzzle around the world. because in those parts of the world where these two layers meet in one place, the Devonian layer lies under the Carboniferous (exceptions are found in places where we can say, based on other evidence, that the rocks were tilted, or even turned upside down). It rarely happens that a complete set of layers is found - from the Cambrian in its lower part to the modern ones at the very top. But since the layers are so recognizable, their relative ages can be determined by building one after another and assembling them like a puzzle around the world. so that a complete set of layers is found - from the Cambrian in its lower part to the modern ones at the very top. But since the layers are so recognizable, their relative ages can be determined by building one after another and assembling them like a puzzle around the world. so that a complete set of layers is found - from the Cambrian in its lower part to the modern ones at the very top. But since the layers are so recognizable, their relative ages can be determined by building one after another and assembling them like a puzzle around the world.

Let's get back to dating. Since the relative order of the named sedimentary layers is well known, and the same order is found all over the world, you can use igneous rocks that lie above or below the sedimentary layers, or which are embedded in them, to date the named sedimentary layers, and therefore fossils inside them. We do not have to look for igneous rocks in the vicinity of a particular fossil to date it. We can say that our fossils belong to, say, the end of the Devonian period, according to their position among the layers. And we know from the radioactive dating of igneous rocks discovered in connection with the Devonian layers around the world that the Devonian period ended about 360 million years ago.
Potassium-argon clocks are just one of many clocks available to geologists who use the same principle on a different time scale. Faster watches, such as carbon-14, work in a slightly different way for an interesting reason, namely, its supplies are constantly replenished. The role of carbon-14 in dating is somewhat different than that of longer-lived isotopes. In particular, what does “reset this watch” mean?
Carbon
Of all the chemical elements, this seems to be the most important for life, without which life on any planet is the most difficult to imagine because of its remarkable ability to form chains, rings and other complex molecular structures. It is introduced into food chains with photosynthesis, a process in which green plants (and some bacteria and animals) absorb carbon dioxide molecules from the atmosphere and use the energy of sunlight to combine carbon atoms with water to create sugars. All carbon in all living things comes, ultimately, through plants, from carbon dioxide in the atmosphere. And he comes back into the atmosphere when we exhale, when we exhale, and when we die.
Most of the carbon in the atmosphere’s carbon dioxide is carbon-12, which is not radioactive. However, approximately one atom per trillion is radioactive carbon-14. It decays quickly enough, with a half-life of 5730 years, as already mentioned, in nitrogen-14. For plant biochemistry, there is no difference between the two isotopes. For plants, carbon is just carbon. Thus, plants incorporate both of these types of carbon atoms into sugars in the same proportion as they are present in the atmosphere. Carbon in the atmosphere (along with the same proportion of atoms 14C) quickly (compared to its half-life) spreads through the food chain when plants are eaten by herbivores, herbivores by predators and so on. All living things, whether plants or animals, have an approximately equal ratio of 14 C / 12 C, which is the same ratio as in the atmosphere.

So when is this watch reset to zero? The moment a living creature, whether it be an animal or a plant, dies. At this moment, it is cut off from the food chain, and from the influx of fresh 14 C. Over the course of centuries, 14 C in the corpse, or piece of wood, or part of the tissue, or other organics, constantly decomposes into nitrogen-14. Therefore, the ratio of 14 C / 12The C in the sample gradually falls below the standard ratio that living things share with the atmosphere. In the end, only 12 C will remain , or rather, the 14 C content will be too small to measure. And the ratio of 14 C / 12 C can be used to calculate the time that has passed since the day of the death of the creature cut off from the food chain and its exchange with the atmosphere.

This is very good, but it only works because there is a continuous replenishment of 14 C in the atmosphere. Without this, a 14 C with a short half-life would long ago have disappeared from the face of the Earth, along with all other natural short-lived isotopes. 14C is special because it is continuously created by cosmic rays bombarding nitrogen atoms in the upper atmosphere.

Nitrogen is the most abundant gas in the atmosphere, and its atomic number is 14, the same as carbon-14. The only difference is that carbon-14 has 6 protons and 8 neutrons, while nitrogen-14 has 7 protons and 7 neutrons (remember, neutrons have almost the same mass as protons). Particles of cosmic rays are able, by bombarding a proton in the nucleus of a nitrogen atom, to turn it into a neutron. When this happens, the atom becomes carbon-14, which is one cell to the left of the nitrogen in the periodic system. The rate of this conversion is approximately constant (depending on fluctuations in solar activity) and therefore radiocarbon dating works. Fortunately, we have an exactcalibration of fluctuations in the supply of 14 C to the atmosphere, and we can adjust them to clarify our age calculations. Remember that, for about the same time range covered by radiocarbon dating, there is an alternative method for wood dating - dendrochronology, which is absolutely accurate for up to a year. Looking at the radiocarbon dates of wooden samples whose age is independently determined by dating using tree rings, we can calibrate this fluctuating error in carbon dating. Now we can use these calibration measurements when we return to organic samples for which we do not have data on tree rings (for most).
Carbon dating is a relatively recent invention and was proposed by Willard Libby.in 1946 (Nobel Prize in Chemistry, 1960). In the early years, substantial amounts of organic material were required for this procedure. Only in the 1970s, a technique called mass spectrometry was adapted for dating, and now only tiny amounts of organic matter are needed. This revolutionized archaeological dating. The most famous example is the Shroud of Turin. Since the face of a bearded man appeared to be imprinted on this notorious piece of fabric in a mysterious way (and, for some reason, for some reason, is in a cylindrical projection), many people hoped that it could happen from the time of Jesus. She first appears in the historical record in the mid-fourteenth century in France, and no one knows where she was before. She has been in Turin since 1578, and in the Vatican since 1983. When mass spectrometry made it possible to date over a tiny shroud sample, rather than a significant piece that would have been needed before, the Vatican allowed a small strip to be cut off. It was divided into three parts and sent to three leading radiocarbon dating laboratories in Arizona, Oxford and Zurich. Working completely independently, without comparing the records, these three laboratories presented their reports on the date when the linen from which the fabric was woven died. The Arizona laboratory pointed to 1304, Oxford to 1200, and Zurich to 1274 AD. All these dates are within error, compatible with each other and with the date of 1350, in which the shroud was first mentioned in history. The dating of the shroud remains controversial, but not for reasons that call into question the very technique of radiocarbon dating. For instance,
There are many different watches that can be used, and they work best on different, but overlapping time scales. A radioactive clock can be used to independently estimate the age of the same piece of rock, if you remember that all the clocks were reset to zero at the same time when this piece of rock crystallized. When such comparisons were made, different watches were compared with each other - within the expected margins of error. This gives great confidence in the correctness of the watch. Thus, mutually calibrated and tested on known rocks, this watch can be safely applied to interesting dating problems, such as the age of the Earth itself. Currently established by Claire Patterson in 1956, an age of 4.55 ± 0.05 billion years is an estimate at which several different hours converge.
The history of establishing the age of the Earth
Just at that time [1946], Harrison Brown of the University of Chicago developed a new method for calculating lead isotopes in rocks of volcanic origin (that is, those that, unlike sedimentary rocks, went through melting). Realizing that the work would be extremely boring, he entrusted it to the young Claire Patterson as a dissertation project. Particularly remarkable is the fact that he promised Patterson that determining the age of the Earth in this new way is a mere trifle. In fact, it will take years.
Patterson began work in 1948. Compared to Thomas Midgley’s breathtaking contribution to human progress, Patterson’s determination of the age of the Earth is more than modest. For seven years - first at the University of Chicago, and then, since 1952, at the California Institute of Technology - he worked in a sterile laboratory, making very accurate measurements of the ratios of lead and uranium in carefully selected samples of ancient rocks.
The difficulty in measuring the age of the Earth was that it required very ancient rocks with crystals, including lead and uranium, about the same age as the planet itself. Younger samples give distortion towards a later time. But rarely really ancient breeds are found on Earth. In the late 1940s, no one fully understood why. In fact, and this is very surprising, only having entered the space age, it was possible to clearly explain where all the ancient rocks went to Earth. (The answer is given by plate tectonics, which, of course, we will get to.) Patterson, meanwhile, had only to try to understand these things, having a very limited set of samples. In the end, he came up with an original idea: it is possible to fill the lack of samples using rocks of extraterrestrial origin.
Patterson put forward a very strong and, as it turned out, correct assumption that many meteorites are the remains of building materials preserved from the early days of the existence of the solar system, and therefore the primary chemical composition could remain more or less intact. Measure the age of these wandering stones - and you will get (with good accuracy) the age of the Earth.
As always, everything was not as simple as it might seem from our cursory description. Meteorites are very rare, and getting their samples is not so easy. In addition, the measurement technique developed by Brown turned out to be extremely complex in detail and required significant refinement. In addition, Patterson’s samples were constantly having problems due to the fact that they were uncontrollably contaminated with large doses of lead contained in the atmosphere every time they got into the air. It was this circumstance that led him to ultimately create a sterile laboratory - the first in the world, according to at least one of the sources.
It took Patterson seven years of hard work to just collect and evaluate samples for final inspection. In the spring of 1953, he brought his samples to the Argonne National Laboratory in Illinois. There he was allotted time on the latest model mass spectrograph - a device capable of detecting and measuring the smallest amount of uranium and lead hidden in ancient crystals. When Patterson finally got the results, he got so excited that he immediately went home to Iowa and asked his mother to put him in the hospital for research, because he thought he had a heart attack.
Soon, at a conference in Wisconsin, Patterson announced the final age of the Earth - 4,550 million years (plus or minus 70 million years) - "a figure that remains unchanged even after fifty years," as McGrain admiringly observes. After two hundred years of trying, the Earth has finally gained age.
Almost immediately Patterson focused on lead-saturating atmosphere. He was amazed to find that even the little that was known about the effects of lead on humans almost invariably turned out to be either false or misleading. And no wonder: for forty years, all studies on the effects of lead were funded exclusively by manufacturers of lead additives.
In one of these studies, a doctor who did not have special training in the field of chemistry related pathology undertook a five-year program during which volunteers were asked to inhale or swallow lead in increasing quantities. Then they tested urine and feces. Unfortunately, the doctor apparently did not know that lead was not released with waste products. On the contrary, it accumulates in the bones and blood - precisely because of this it is so dangerous - but neither the bones nor the blood were tested. As a result, lead was issued a certificate of complete harmlessness to health.
Patterson quickly determined that he was in the atmosphere — and actually remained today because he was not going anywhere — a huge amount of lead. And about 90% of it seems to have come out of the exhaust pipes of cars. But he could not prove it. He needed to find a way to compare the current level of lead in the atmosphere with the level that existed until 1923, when the commercial production of tetraethyl lead began. And then he realized that glaciers could give an answer.
It was known that in places like Greenland, snow fell is deposited in separate layers (due to seasonal temperature fluctuations, slight changes in their color from winter to summer are observed). By counting these layers and measuring the amount of lead in each of them, Patterson could calculate the concentration of lead in the earth's atmosphere at any time over hundreds or even thousands of years. This idea formed the basis for the study of glacial cores, on which modern climatology is largely based.
Patterson discovered that until 1923 there was almost no lead in the atmosphere, and after that the level of lead steadily and dangerously crawled up. Now his life’s business was to remove lead from gasoline. He became a constant and often harsh critic of the industrial production of lead and the interests behind it.
The campaign turned out to be devilishly difficult. Ethyl was a powerful global corporation and had many senior friends. (Among its directors were Supreme Court member Lewis Powell and Gilbert Grovernor of the National Geographic Society.) Patterson suddenly discovered that funds for his scientific research were either withdrawn or allocated with great difficulty. The American Petroleum Institute terminated the research contract with him, the United States Health Service, which was considered an impartial government body, too.
As Patterson increasingly became a hindrance to his academic institution, lead company officials often clicked on the trustees of the California Institute of Technology to silence him or leave. According to Jamie Lincoln Kitman, who wrote in The Nation in 2000, Ethil’s management offered to donate funds to support the department’s institute if Patterson left. It got to the point of absurdity when he, undoubtedly, America’s most prominent atmospheric lead expert, was removed from the Lead Pollution Commission of the National Research Council in 1971.
We must pay tribute to Patterson, he remained firm in his convictions. And ultimately, his efforts led to the adoption of the Clean Air Act 1970, and in 1986 to the complete withdrawal of leaded gasoline from the United States. Almost immediately, the lead content in the blood of Americans fell by 80%. But because lead remains forever, every modern American has 625 times more lead in his blood than his fellow tribesman who lived a hundred years ago. The lead content in the atmosphere also continues to increase, and quite legitimately, by about one hundred thousand tons per year, mainly due to its production, smelting and industrial processing. The United States also banned the use of lead in indoor painting. “44 years after most of Europe,” says McGrain. Amazing that
As for Ethyl Corporation, it still thrives, although General Motor, Standard Oil and DuPont are no longer involved. (In 1962, they sold their shares to Alberger Paper.) According to McGrain, back in February 2001, Ethil continued to claim "that studies have not confirmed that leaded gasoline poses a risk to human health or the environment." There is no mention of Thomas Midgley , the inventor of tetraethyl lead, on her site in the company's history , but simply contains a link to the original product containing “certain chemical compounds”.
Ethyl no longer produces leaded gasoline, although according to company reports for 2001, the sale of tetraethyl lead in 2000 still earned it $ 25.1 million (out of a total of $ 795 million), even more than in 1999 ( 24.1 million dollars), but less than in 1998 (117 million dollars). In its report, the company declares its determination to "maximize revenues from sales of tetraethyl lead, the use of which continues to decline gradually in the world." "Ethyl" sells tetraethyl lead around the world in agreement with the British company Associated Octel Ltd.
As for the other punishment that Thomas Midgley left for us - chlorofluorocarbons, they were banned in the United States in 1974, but these insidious invisibles are terribly tenacious, and those that got into the atmosphere earlier (for example, as part of deodorants or hair sprays), they will almost certainly remain there and eat ozone long after you and I are gone. Even worse, every year we continue to add a huge amount of chlorofluorocarbons to the atmosphere. According to Wayne Biddle, the market annually gets 27 million kilograms of this potion worth one and a half billion dollars. So who produces it? We, that is, many of our large corporations producing it at our foreign enterprises. In third world countries it will not be banned until 2010.
Claire Patterson died in 1995. He did not receive the Nobel Prize for his labors. Geologists do not give it. It is even stranger that half a century of hard, selfless work did not bring him either glory or little recognition. One could argue that he was the most influential geologist of the twentieth century. However, who has heard of Claire Patterson? Most geology textbooks do not mention it. In two recent popular books on the history of determining the age of the Earth, they managed to distort his name.
In early 2001, a reviewer of one of these books in Nature magazine made another startling mistake by introducing Patterson as a woman.
Be that as it may, thanks to the work of Patterson, the Earth finally reached an age by 1953, with which everyone could agree.
Patterson began work in 1948. Compared to Thomas Midgley’s breathtaking contribution to human progress, Patterson’s determination of the age of the Earth is more than modest. For seven years - first at the University of Chicago, and then, since 1952, at the California Institute of Technology - he worked in a sterile laboratory, making very accurate measurements of the ratios of lead and uranium in carefully selected samples of ancient rocks.
The difficulty in measuring the age of the Earth was that it required very ancient rocks with crystals, including lead and uranium, about the same age as the planet itself. Younger samples give distortion towards a later time. But rarely really ancient breeds are found on Earth. In the late 1940s, no one fully understood why. In fact, and this is very surprising, only having entered the space age, it was possible to clearly explain where all the ancient rocks went to Earth. (The answer is given by plate tectonics, which, of course, we will get to.) Patterson, meanwhile, had only to try to understand these things, having a very limited set of samples. In the end, he came up with an original idea: it is possible to fill the lack of samples using rocks of extraterrestrial origin.
Patterson put forward a very strong and, as it turned out, correct assumption that many meteorites are the remains of building materials preserved from the early days of the existence of the solar system, and therefore the primary chemical composition could remain more or less intact. Measure the age of these wandering stones - and you will get (with good accuracy) the age of the Earth.
As always, everything was not as simple as it might seem from our cursory description. Meteorites are very rare, and getting their samples is not so easy. In addition, the measurement technique developed by Brown turned out to be extremely complex in detail and required significant refinement. In addition, Patterson’s samples were constantly having problems due to the fact that they were uncontrollably contaminated with large doses of lead contained in the atmosphere every time they got into the air. It was this circumstance that led him to ultimately create a sterile laboratory - the first in the world, according to at least one of the sources.
It took Patterson seven years of hard work to just collect and evaluate samples for final inspection. In the spring of 1953, he brought his samples to the Argonne National Laboratory in Illinois. There he was allotted time on the latest model mass spectrograph - a device capable of detecting and measuring the smallest amount of uranium and lead hidden in ancient crystals. When Patterson finally got the results, he got so excited that he immediately went home to Iowa and asked his mother to put him in the hospital for research, because he thought he had a heart attack.
Soon, at a conference in Wisconsin, Patterson announced the final age of the Earth - 4,550 million years (plus or minus 70 million years) - "a figure that remains unchanged even after fifty years," as McGrain admiringly observes. After two hundred years of trying, the Earth has finally gained age.
Almost immediately Patterson focused on lead-saturating atmosphere. He was amazed to find that even the little that was known about the effects of lead on humans almost invariably turned out to be either false or misleading. And no wonder: for forty years, all studies on the effects of lead were funded exclusively by manufacturers of lead additives.
In one of these studies, a doctor who did not have special training in the field of chemistry related pathology undertook a five-year program during which volunteers were asked to inhale or swallow lead in increasing quantities. Then they tested urine and feces. Unfortunately, the doctor apparently did not know that lead was not released with waste products. On the contrary, it accumulates in the bones and blood - precisely because of this it is so dangerous - but neither the bones nor the blood were tested. As a result, lead was issued a certificate of complete harmlessness to health.
Patterson quickly determined that he was in the atmosphere — and actually remained today because he was not going anywhere — a huge amount of lead. And about 90% of it seems to have come out of the exhaust pipes of cars. But he could not prove it. He needed to find a way to compare the current level of lead in the atmosphere with the level that existed until 1923, when the commercial production of tetraethyl lead began. And then he realized that glaciers could give an answer.
It was known that in places like Greenland, snow fell is deposited in separate layers (due to seasonal temperature fluctuations, slight changes in their color from winter to summer are observed). By counting these layers and measuring the amount of lead in each of them, Patterson could calculate the concentration of lead in the earth's atmosphere at any time over hundreds or even thousands of years. This idea formed the basis for the study of glacial cores, on which modern climatology is largely based.
Patterson discovered that until 1923 there was almost no lead in the atmosphere, and after that the level of lead steadily and dangerously crawled up. Now his life’s business was to remove lead from gasoline. He became a constant and often harsh critic of the industrial production of lead and the interests behind it.
The campaign turned out to be devilishly difficult. Ethyl was a powerful global corporation and had many senior friends. (Among its directors were Supreme Court member Lewis Powell and Gilbert Grovernor of the National Geographic Society.) Patterson suddenly discovered that funds for his scientific research were either withdrawn or allocated with great difficulty. The American Petroleum Institute terminated the research contract with him, the United States Health Service, which was considered an impartial government body, too.
As Patterson increasingly became a hindrance to his academic institution, lead company officials often clicked on the trustees of the California Institute of Technology to silence him or leave. According to Jamie Lincoln Kitman, who wrote in The Nation in 2000, Ethil’s management offered to donate funds to support the department’s institute if Patterson left. It got to the point of absurdity when he, undoubtedly, America’s most prominent atmospheric lead expert, was removed from the Lead Pollution Commission of the National Research Council in 1971.
We must pay tribute to Patterson, he remained firm in his convictions. And ultimately, his efforts led to the adoption of the Clean Air Act 1970, and in 1986 to the complete withdrawal of leaded gasoline from the United States. Almost immediately, the lead content in the blood of Americans fell by 80%. But because lead remains forever, every modern American has 625 times more lead in his blood than his fellow tribesman who lived a hundred years ago. The lead content in the atmosphere also continues to increase, and quite legitimately, by about one hundred thousand tons per year, mainly due to its production, smelting and industrial processing. The United States also banned the use of lead in indoor painting. “44 years after most of Europe,” says McGrain. Amazing that
As for Ethyl Corporation, it still thrives, although General Motor, Standard Oil and DuPont are no longer involved. (In 1962, they sold their shares to Alberger Paper.) According to McGrain, back in February 2001, Ethil continued to claim "that studies have not confirmed that leaded gasoline poses a risk to human health or the environment." There is no mention of Thomas Midgley , the inventor of tetraethyl lead, on her site in the company's history , but simply contains a link to the original product containing “certain chemical compounds”.
Ethyl no longer produces leaded gasoline, although according to company reports for 2001, the sale of tetraethyl lead in 2000 still earned it $ 25.1 million (out of a total of $ 795 million), even more than in 1999 ( 24.1 million dollars), but less than in 1998 (117 million dollars). In its report, the company declares its determination to "maximize revenues from sales of tetraethyl lead, the use of which continues to decline gradually in the world." "Ethyl" sells tetraethyl lead around the world in agreement with the British company Associated Octel Ltd.
As for the other punishment that Thomas Midgley left for us - chlorofluorocarbons, they were banned in the United States in 1974, but these insidious invisibles are terribly tenacious, and those that got into the atmosphere earlier (for example, as part of deodorants or hair sprays), they will almost certainly remain there and eat ozone long after you and I are gone. Even worse, every year we continue to add a huge amount of chlorofluorocarbons to the atmosphere. According to Wayne Biddle, the market annually gets 27 million kilograms of this potion worth one and a half billion dollars. So who produces it? We, that is, many of our large corporations producing it at our foreign enterprises. In third world countries it will not be banned until 2010.
Claire Patterson died in 1995. He did not receive the Nobel Prize for his labors. Geologists do not give it. It is even stranger that half a century of hard, selfless work did not bring him either glory or little recognition. One could argue that he was the most influential geologist of the twentieth century. However, who has heard of Claire Patterson? Most geology textbooks do not mention it. In two recent popular books on the history of determining the age of the Earth, they managed to distort his name.
In early 2001, a reviewer of one of these books in Nature magazine made another startling mistake by introducing Patterson as a woman.
Be that as it may, thanks to the work of Patterson, the Earth finally reached an age by 1953, with which everyone could agree.
Criticism
So, adherents of the "alternative history" can say, for example, that something is amiss with the potassium-argon clock. What if the modern, very low decay rate of 40 K acted only after the Noah Flood? If before it the half-life of 40 K was radically different, and was, for example, several centuries, and not 1.26 billion years? A special reservation in such a statement is striking. Why should the laws of physics change that way, Ad Hoc- so convenient and so large? It looks even more flashy if you need to make special mutually agreed reservations for each of the watches separately. Currently, all applied methods are consistent with each other in determining the date of formation of the Earth in the range between four and five billion years ago. And they are based on the assumption that the half-life is always the same, which we fix today, as the well-known laws of physics directly prescribe them to be. Deniers of history should play around with the half-life of all isotopes in their different proportions so that they all agree with the assumption that the Earth was formed 6,000 years ago. This is what I call a special disclaimer. Some other methods are not even mentioned here, for example, “track dating”, which also leads to the same result. One should take into account the huge differences in the time scales of different clocks, think about the degree of tension and the difficulty of fitting the laws of physics, which would be necessary to make all the clocks fit together in a range of several orders of magnitude, which is 6,000 years old for the Earth, not 4,55 billion ! Given that the only motive for such a fit is the desire to uphold the creation myth of a private group of Bronze Age tribes, it is not surprising that mostly ignorant people buy it.
However, there are always errors. Buried organics can be contaminated with extraneous carbon, both “ancient” (with a low proportion of 14 C) and “young”. As a result, there arise, respectively, “aging errors” and “rejuvenation errors”. In addition, the ratio of 14 C / 12 C in the atmosphere is not constant. For example, human economic activity and especially nuclear testing strongly affect this value. The rate of 14 C formation in the upper atmosphere depends on the intensity of cosmic and solar radiation, and these are variables. The ratio of 14 C / 12 C depends on the total concentration of CO 2in the atmosphere, the composition of which is also changing. All these natural fluctuations, however, are not very large and they are taken into account. A truly serious problem is only the possibility of contamination of the sample with extraneous carbon. Indeed, accuracy depends on “people in the field” and on the laboratory assistant. It is here that they try to compromise science, saying: “Scientists have determined the age of a live sheep at 15,000 years!”, Silent about the incorrect methodology - a sample could be taken from an animal grazing near the highway. And carbon got into plants from automobile exhausts, which, burning oil products, release carbon from long-dead organisms.
As for the "Mesozoic hammer", "the chain in hard coal", "trilobite crushed by the boot" - when assessing the degree of reliability of such "news" on your own, you need to keep in mind that there should be a link to an article that describes in detail - where, when, by whom and under what circumstances was the find made. Is it made by the scientist himself? There should be an archaeological context: a layer, which objects were nearby and so on.
Information obtained through a chain of “testimonies” is the most inaccurate information (although it is sometimes accepted in courts). A typical example of such a distortion of information is the children's game “broken phone”. Not to mention our imperfect perception and insecure memory.
Check the clock
The inaccuracy of most methods of absolute geochronology does not give grounds to deny the validity of dating in archeology, paleontology and evolutionary biology (as, for example, advocates of creationism, Fomenko’s “New Chronology” and other pseudoscientific concepts do). The main advantage of these methods is that there are many of them. And in the vast majority of cases, they nevertheless give similar results, which, moreover, are remarkably consistent with the data of relative geochronology (the order of the arrangement of geological layers). If this were not so, there would be nothing to talk about! It’s like with ship’s chronometers: if he’s alone, you can’t determine when he’s lying; if there are two of them, you can already understand that one of them is lying, it is not clear which of the two, and if three or more, the exact time can be found almost always.
For this reason, in scientific research, it is customary to determine the age of objects today using several independent methods. If this rule is violated, the result looks controversial for most specialists.
Finally, I apologize for the somewhat “captain's” style of presentation - it turned out that
Literature
1. Richard Dawkins' The most spectacular show on Earth "
2. Website" Elements "
3. Bill Bryson's" A Short History of Nearly Everything "
4. Wikipedia