Expert opinion: The future of metal glasses
Today we will tell you about the future of metal glasses. To do this, we turned to our leading scientist, international expert in the field of bulk metal glasses, doctor of technical sciences, professor at Tohoku University / Japan, chief researcher, head of the laboratory of the Institute for Advanced Materials Research and project manager Metastable two-phase metal materials with high specific strength at NITU “MISiS” - to Dmitry Valentinovich Luzgin .
This project has no analogues both in Russia and abroad, given the extensive nature of research, the range of properties studied and the types of materials used. The competence of the scientist is confirmed by scientific articles in journals such asNature, Nanoscale, Acta Materialia, Advanced Functional Materials, Scientific Reports, Applied Physics Letters , as well as peer-reviewed journal Nature Communications, Acta Materialia, Applied Physics Letters, Journal of Materials Research, Materials Science and Engineering, Journal of Non-crystalline Solids, Journal of Materials Science.
A fascinating scientific story about steel and alloys awaits you! In his expert opinion, Dmitry Valentinovich discusses the future of metal glasses, their application, ways to improve their mechanical properties and prospects for use in specific areas.
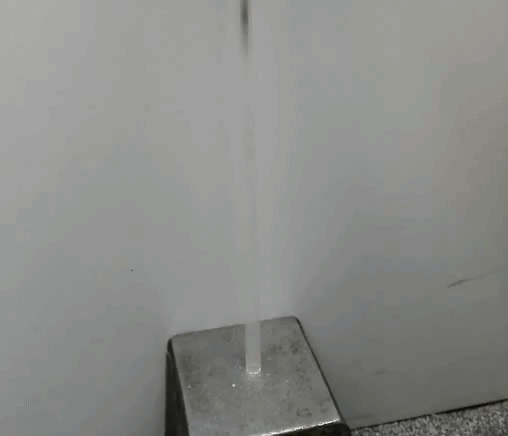
Since the end of the last century, numerous experimental and theoretical studies conducted by scientists provide a small improvement in the complex of mechanical properties of metal alloys (especially specific: per unit mass) using classical methods: solid solution hardening, hardening, aging, plastic deformation, annealing, etc. . And if in heavy engineering, shipbuilding (except for small boats), the automotive industry, steel and aluminum alloys are and will be out of competition, in civil aviation and sports equipment, metals are significantly replaced by non-metallic composite materials. For example, a Boeing 787 is mainly made of carbon fiber reinforced polymer composites (50% by weight, compared to 12% in a Boeing 777) [1].
Fundamentally new methods for producing and processing alloys are necessary to create a new generation of structural and functional metal materials.
Industrial metal alloys harden to form a crystalline structure even at high cooling rates. The preparation of amorphous / glassy metal alloys (or metal glasses), including pure metals, requires ultrahigh cooling rates, for example, when thin films are sprayed onto a cooled substrate from the gaseous phase [2]. The sputtering of pure metals into nanoscale droplets leads not only to high cooling rates, but also to a low probability of the appearance of a critical nucleus of a crystalline phase in a nanovolume [3].
Alloys with a higher tendency to vitrification, called the glass-forming ability, usually of eutectic composition, have been obtained since the 60s of the last century by rapid cooling of the melt at speeds of the order of 1 MK / s per rotating copper disk or by compressing a drop of melt between two metal planes [ 4]. In this case, metal alloys continuously undergo a glassy state upon cooling and undergo a reverse transformation upon subsequent heating with a sufficiently high heating rate.
In the 70s of the last century, the first macroscopic castings of amorphous palladium alloys with a size of the order of 1 mm were obtained in each of the 3 spatial dimensions, which were later called bulk metal glasses [5]. More massive samples were obtained in the 80s after flux treatment of the melt, which made it possible to suppress the heterogeneous nucleation of crystals [6], but because of the exceptional high cost of the main component of palladium, for a long time they were not of particular interest to scientists and engineers.
In the 90s, bulk metal glasses (OMS) [7] with a size> 1 mm in each of the 3 spatial dimensions ( Fig. 1 ) were obtained on the basis of widely used metals: magnesium, titanium, copper, iron, etc. in double, triple, quadruple and multicomponent alloys.
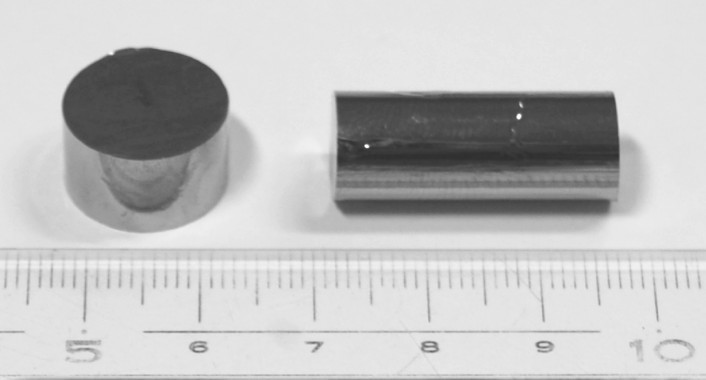
A statistical analysis of the available information on OMS showed an increase in their glass-forming ability from double to triple and quadruple alloys. Also, when analyzing a database of 95 ternary alloys with a known glass-forming ability in the form of a critical OMC diameter (flux-treated alloys were not taken into account), a statistical regularity was found in the distribution of OMC compositions ( Fig. 2) [8]. The local maxima of the critical diameter were located near the compositions A70B20C10, A65B25C10, A65B20C15, A56B32C12, A55B28C17, A44B43C13 and A44B38C18, and the local minima near the compositions A75B20C5, A75B15C10, A60B35C5, A55B35C15, A55B35C15, A55B35C15. This indicates the regularities in the composition of the OMC and the nonrandomness of atomic ratios. Alloy A50B25C25 corresponds to compound A2BC, A60B35C5 to compound A3 (B + C) 2, and A75B20C5 and A75B15C10 are close to the pseudo-double compound A3 (B + C).
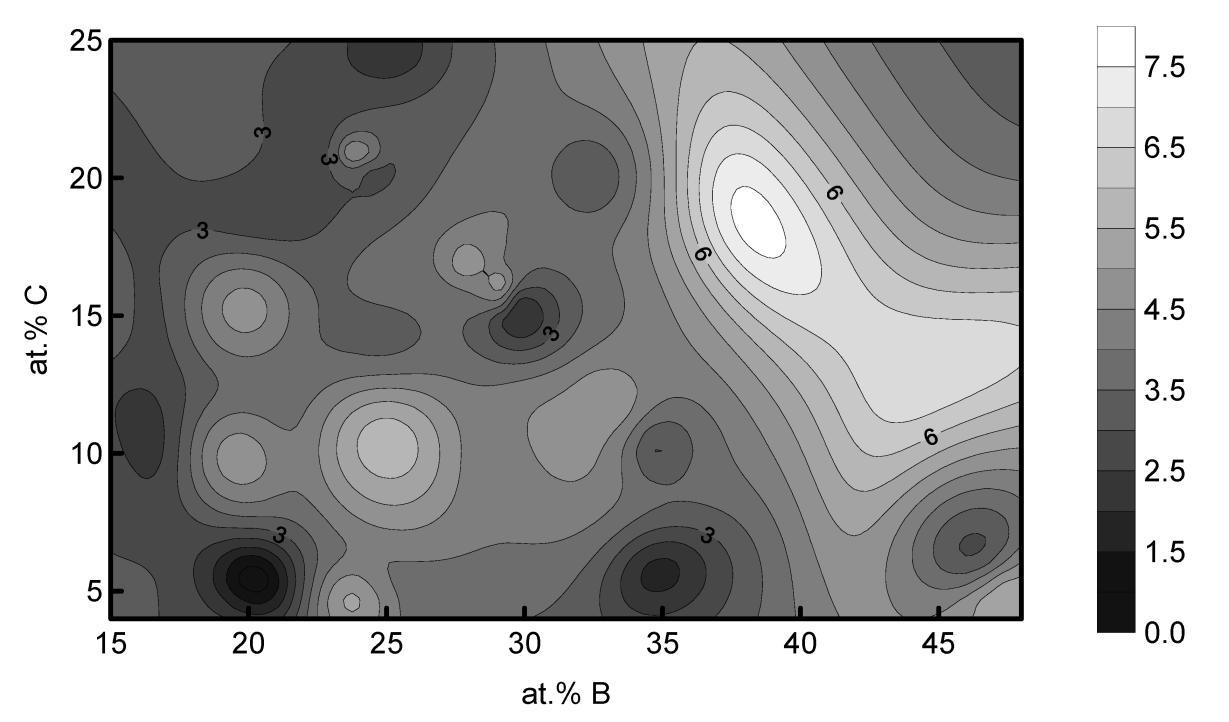
The atomic structure of glasses demonstrating the absence of long-range order in the arrangement of atoms ( Fig. 3) determine their properties, in particular mechanical. In terms of strength and specific strength, they significantly exceed the corresponding crystalline alloys due to the impossibility of using the mechanisms of accommodational deformation of a dislocation or twin type. The conditional yield stress of OMS reaches ~ 2 GPa for OMS based on Cu, Ti and Zr, ~ 3 GPa based on Ni, ~ 4 GPa based on Fe, ~ 5 GPa based on Fe and Co, and 6 GPa for cobalt alloys. The structure of metal glass also provides elastic deformation of up to 2%, which in combination with a high yield strength leads to large values of the stored energy of elastic deformation (indicators σy2 / E and σy2 / ρЕ, where σy, ρ and Е are the yield strength, density and Young's modulus, respectively). It should be noted that recent studies indicate the presence of atomic clusters in the OMC [9].
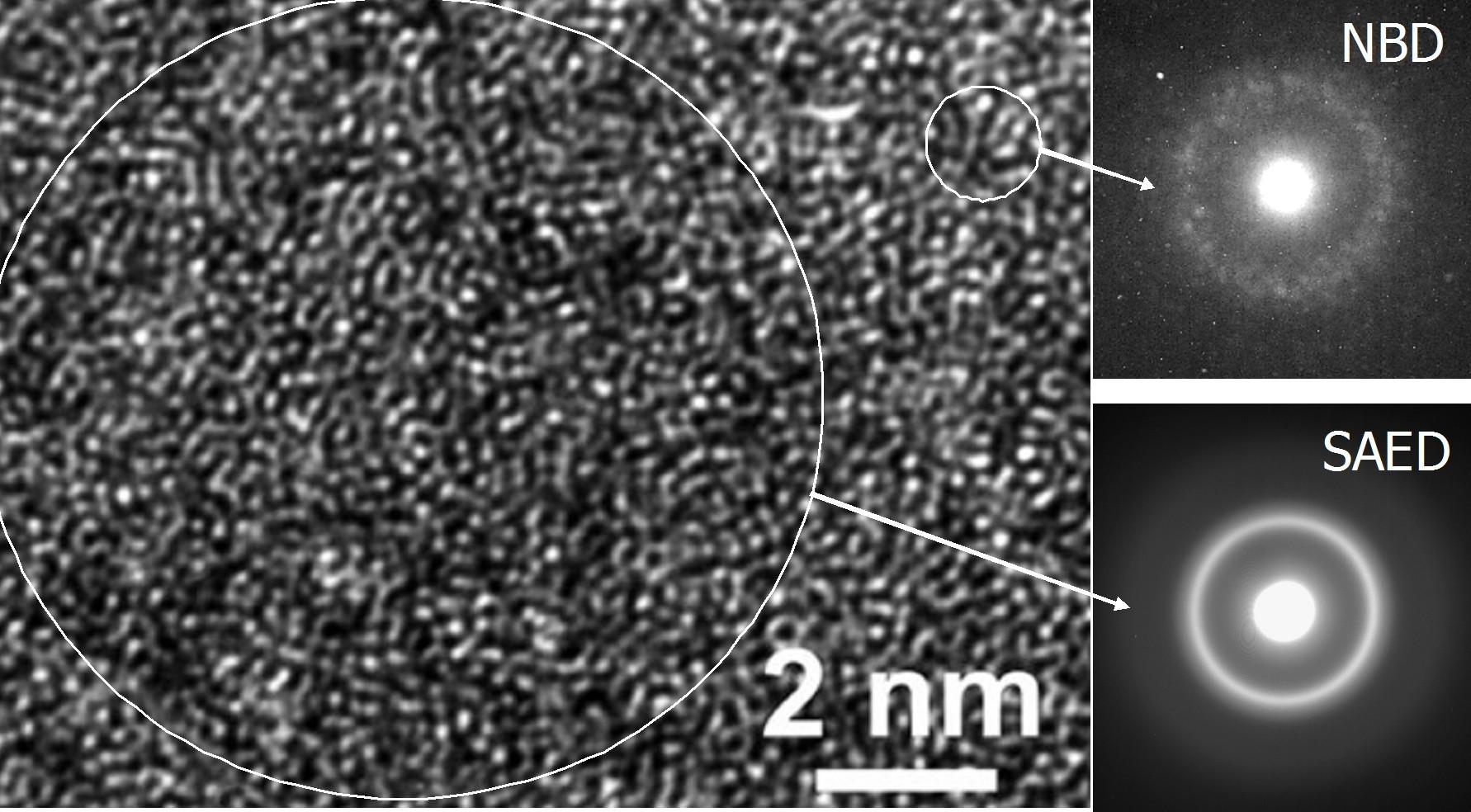
OMSs possess not only high strength, hardness, wear resistance and high values of elastic deformation before the onset of plastic deformation, but also high corrosion resistance, including spontaneous passivation in some solutions. High hardness, wear resistance, surface quality of the OMC, as well as fluidity during heating determines their use in micromachines as transmission mechanisms (gears), components of high-precision mechanical systems. OMSs based on iron and cobalt with saturation magnetization up to 1.5 T have record low values of coercive force less than 1 A / m and are actively used as soft magnetic materials. It should be noted that in Russia metal scientists based on iron and cobalt were engaged in such scientists as A.M. Glezer, S.D. Kaloshkin and many others.
The glass transition phenomenon observed during the transition from liquid to glass and devitrification upon heating is one of the most important problems of solid state physics that have not been completely resolved. Namely, are the amorphous and liquid phases the same phase, only observed at different temperatures, or is there a phase transition from the liquid state to the amorphous state and vice versa, and if so, what kind of phase transition is this? Some successes have been achieved using computer simulation, but there is still no complete clarity.
For a long time, it was not clear where such a property of “superfluid” metal comes from: “brittleness”: a strong deviation of the temperature dependence of its viscosity from the Arrhenius law, while the viscosity of an equilibrium liquid above the liquidus temperature (Tl) follows this law. The author and colleagues did not show in-situ X-ray diffraction by synchrotron radiation that the Pd42.5Cu30Ni7.5P20 alloy was actively formed in the atomic structure near the glass transition temperature of metal clusters covalently bound to P, which correlated with the temperature dependence of the viscosity of the liquid [10] upon cooling melt, as schematically shown in Fig. 4 . Note that the viscosity of a liquid when cooled to Tg changes by 10 orders of magnitude.
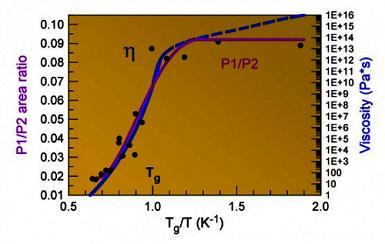
The OMC perfectly and uniformly deform plastically when heated to a region of supercooled liquid before crystallization (above Tg, but below Tx — crystallization temperature of the supercooled liquid [11,12]), and can be used as models for dies ( Fig. 5 ).
Traditional methods of processing metals by pressure at a low cost of alloy components often require expensive processing steps of the source block to obtain the final product. This leads to a large amount of waste material. In the case of OMS, by means of rapid heating to the region of supercooled liquid, it is possible to obtain a product with high surface quality in one stage, as in superplastic molding. But, due to the absence of grain boundaries, OMS will be preferable for microobjects than superplastic alloys due to the extremely high surface quality.
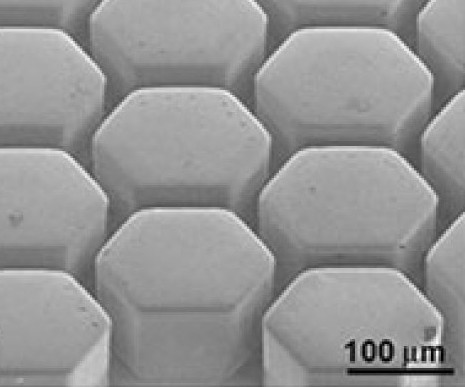
Nevertheless, one of the main obstacles to the wider use of glassy alloys is their limited ductility at room temperature [13]. Many OMS are destroyed before the start of macroplastic deformation during the formation of one shear band.
This is due to softening of the sample in local shear deformation bands (bands 10–20 nm thick, in which the material begins to flow under the action of high local stresses) and further localization of deformation in these bands, in contrast to crystalline alloys in which strain hardening leads to more uniform deformation in multiple slip bands. However, if several shear bands with alternating localization of deformation arise ( Fig. 6), then up to a certain point, macroscopically uniform deformation of the sample can occur, which is preferable for obtaining more plastic OMCs [14]. In this regard, the study of the process of nucleation and propagation of shear bands in the OMC is of great interest. As a result of improved compositions, record high fracture toughness values of the order of 100 MPa√m were obtained for the Zr61Ti2Cu25Al12 [15] and Pd79Ag3.5P6Si9.5Ge2 [16] OMCs, as well as alloys of hypereutectic compositions that are not embrittled during structural relaxation [17]. The recently proposed method of cyclic treatment in liquid nitrogen, due to the difference in the thermal expansion coefficients of atomic clusters with high and low densities, leads to the “rejuvenation” of metal glass (its transition to an increased energy state) and its plasticity [18].
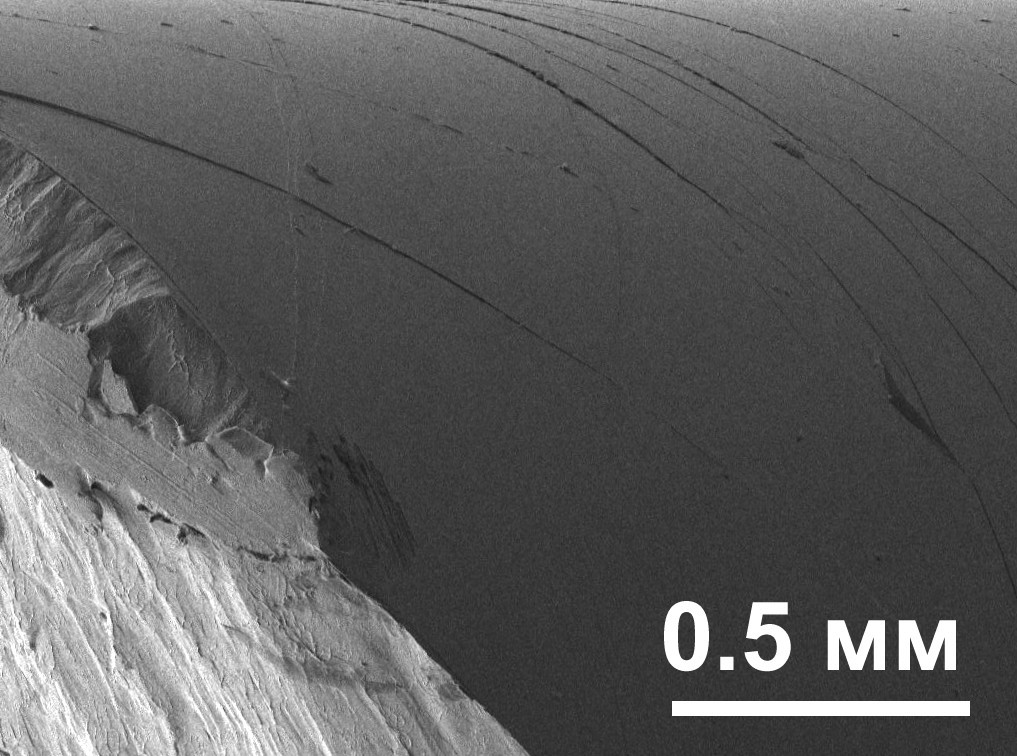
Today, two-phase materials (composite type) are becoming more widespread, since they are able to combine, in an optimal ratio, the required set of operational properties. MHIs have uniquely high strengths, but, as a rule, lack of ductility, especially tensile. Crystalline alloys have high ductility, and polymeric materials also have low density. Therefore, the creation of a new class of light and durable two-phase materials based on metal glass and a crystal (or polymer) helps to solve the problem associated with improving mechanical structural materials, i.e. to obtain a material with a uniquely high rate of specific strength and ductility.Fig. 7 ).
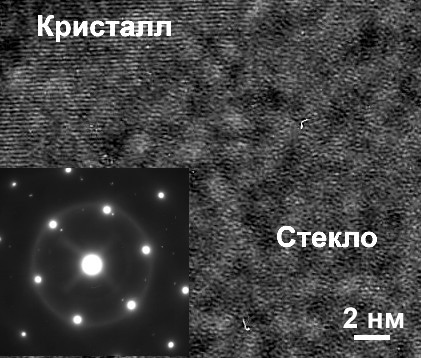
A promising material is nanostructured metal glasses ( Fig. 8 ) recently obtained in collaboration with the ICM Department , which can be used as materials for medicine (high corrosion resistance and good cell adhesion) and catalysis (developed surface) [21].
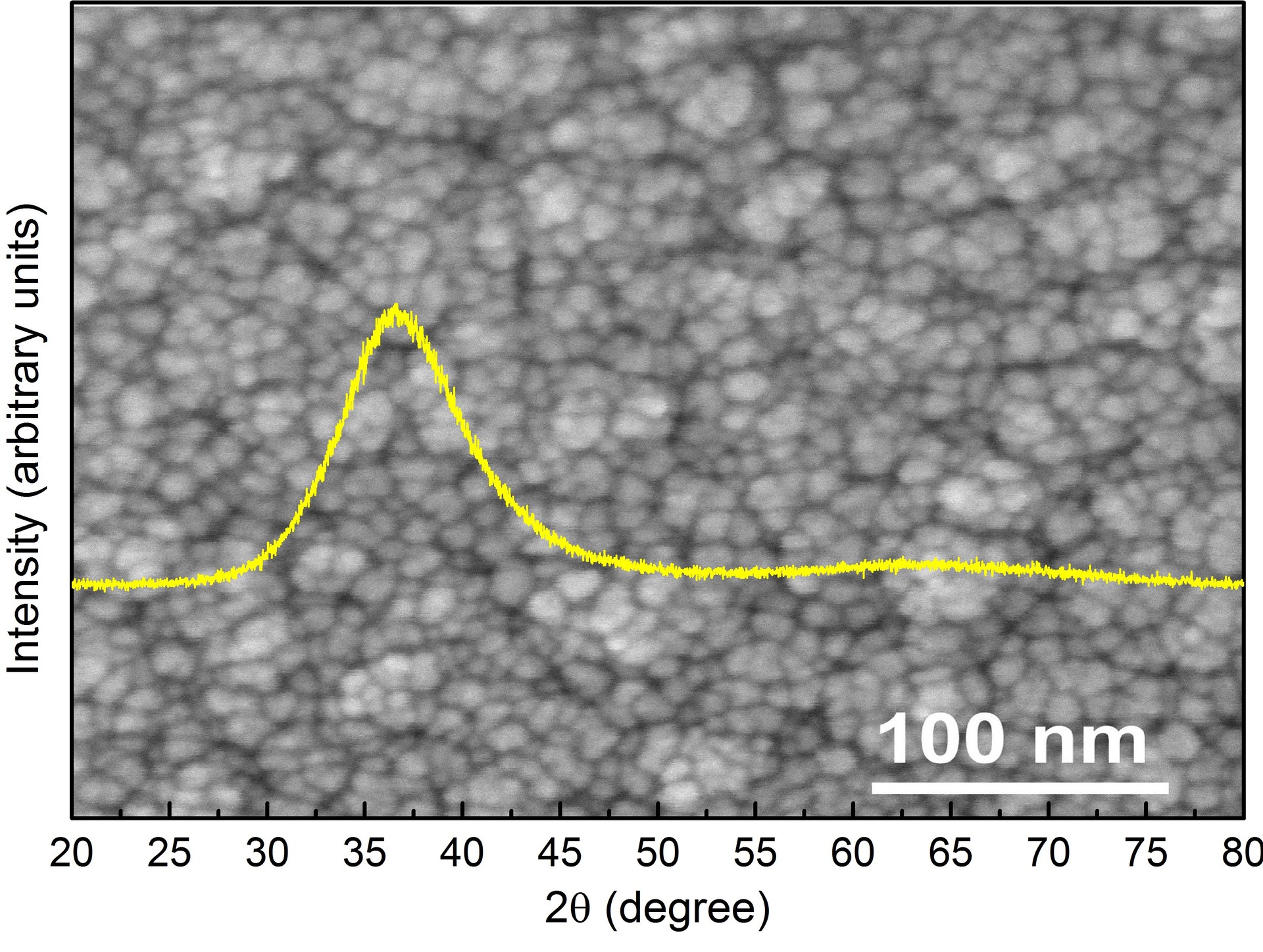
It has recently been shown that the homogeneous amorphous oxides formed on the surface of the Ni-Nb OMC have an amorphous structure without defects in the form of grain boundaries inherent in crystals, through which an electric current leak can pass. They demonstrate semiconductor properties, and their conductivity changes from electron to hole after annealing [22]. This material corresponds to a Schottky diode with a very low reverse current, the conductivity of which can be controlled by annealing in oxygen ( Fig. 9 ).

In conclusion, it should be noted that metal glasses after a half-century history are still of great interest for studying their unusual properties and structure, and biphasic glass-crystal materials are very promising for practical use as high-strength structural materials in areas where the cost is slightly higher material is not a big deal. Of course, one should not expect that OMC and two-phase materials, even when the compositions are cheaper, will replace structural steels or aluminum alloys in construction and heavy engineering. However, they, and two-phase materials such as glass-crystal, are already finding wider application in certain areas where they are superior to competitors: orthopedic screws in medicine (biocompatibility), micromachines (formability, wear resistance),
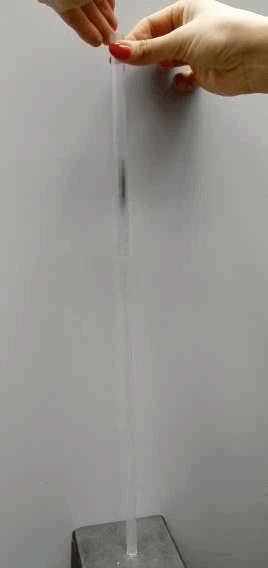
Fig. 10 This example illustrates the high ability of the OMC to store the energy of elastic deformation when the ingot falls from a certain height in the tube to the anvil.
This project has no analogues both in Russia and abroad, given the extensive nature of research, the range of properties studied and the types of materials used. The competence of the scientist is confirmed by scientific articles in journals such asNature, Nanoscale, Acta Materialia, Advanced Functional Materials, Scientific Reports, Applied Physics Letters , as well as peer-reviewed journal Nature Communications, Acta Materialia, Applied Physics Letters, Journal of Materials Research, Materials Science and Engineering, Journal of Non-crystalline Solids, Journal of Materials Science.
A fascinating scientific story about steel and alloys awaits you! In his expert opinion, Dmitry Valentinovich discusses the future of metal glasses, their application, ways to improve their mechanical properties and prospects for use in specific areas.
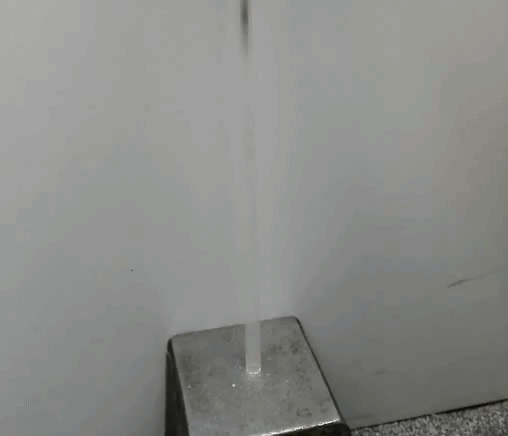
The full version of the animation with a description at the end of the publication.
![]() | Dmitry Valentinovich Luzgin Doctor of Technical Sciences Professor, Chief Researcher, Head. Laboratory of Tohoku University / Japan, Project Manager at NUST "MISiS" |
Since the end of the last century, numerous experimental and theoretical studies conducted by scientists provide a small improvement in the complex of mechanical properties of metal alloys (especially specific: per unit mass) using classical methods: solid solution hardening, hardening, aging, plastic deformation, annealing, etc. . And if in heavy engineering, shipbuilding (except for small boats), the automotive industry, steel and aluminum alloys are and will be out of competition, in civil aviation and sports equipment, metals are significantly replaced by non-metallic composite materials. For example, a Boeing 787 is mainly made of carbon fiber reinforced polymer composites (50% by weight, compared to 12% in a Boeing 777) [1].
Fundamentally new methods for producing and processing alloys are necessary to create a new generation of structural and functional metal materials.
Industrial metal alloys harden to form a crystalline structure even at high cooling rates. The preparation of amorphous / glassy metal alloys (or metal glasses), including pure metals, requires ultrahigh cooling rates, for example, when thin films are sprayed onto a cooled substrate from the gaseous phase [2]. The sputtering of pure metals into nanoscale droplets leads not only to high cooling rates, but also to a low probability of the appearance of a critical nucleus of a crystalline phase in a nanovolume [3].
Alloys with a higher tendency to vitrification, called the glass-forming ability, usually of eutectic composition, have been obtained since the 60s of the last century by rapid cooling of the melt at speeds of the order of 1 MK / s per rotating copper disk or by compressing a drop of melt between two metal planes [ 4]. In this case, metal alloys continuously undergo a glassy state upon cooling and undergo a reverse transformation upon subsequent heating with a sufficiently high heating rate.
In the 70s of the last century, the first macroscopic castings of amorphous palladium alloys with a size of the order of 1 mm were obtained in each of the 3 spatial dimensions, which were later called bulk metal glasses [5]. More massive samples were obtained in the 80s after flux treatment of the melt, which made it possible to suppress the heterogeneous nucleation of crystals [6], but because of the exceptional high cost of the main component of palladium, for a long time they were not of particular interest to scientists and engineers.
In the 90s, bulk metal glasses (OMS) [7] with a size> 1 mm in each of the 3 spatial dimensions ( Fig. 1 ) were obtained on the basis of widely used metals: magnesium, titanium, copper, iron, etc. in double, triple, quadruple and multicomponent alloys.
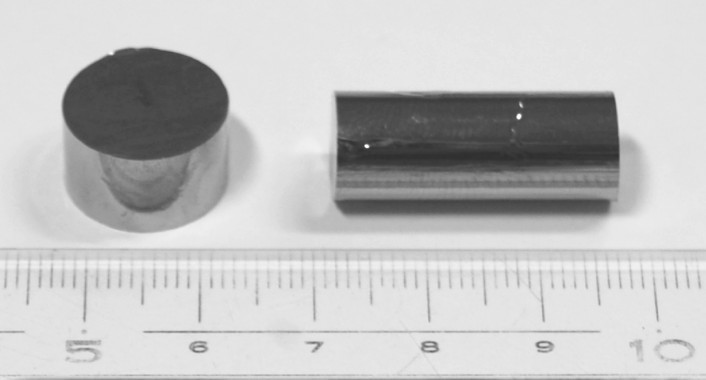
Fig. 1. Samples of the OMS castings (optical image).
A statistical analysis of the available information on OMS showed an increase in their glass-forming ability from double to triple and quadruple alloys. Also, when analyzing a database of 95 ternary alloys with a known glass-forming ability in the form of a critical OMC diameter (flux-treated alloys were not taken into account), a statistical regularity was found in the distribution of OMC compositions ( Fig. 2) [8]. The local maxima of the critical diameter were located near the compositions A70B20C10, A65B25C10, A65B20C15, A56B32C12, A55B28C17, A44B43C13 and A44B38C18, and the local minima near the compositions A75B20C5, A75B15C10, A60B35C5, A55B35C15, A55B35C15, A55B35C15. This indicates the regularities in the composition of the OMC and the nonrandomness of atomic ratios. Alloy A50B25C25 corresponds to compound A2BC, A60B35C5 to compound A3 (B + C) 2, and A75B20C5 and A75B15C10 are close to the pseudo-double compound A3 (B + C).
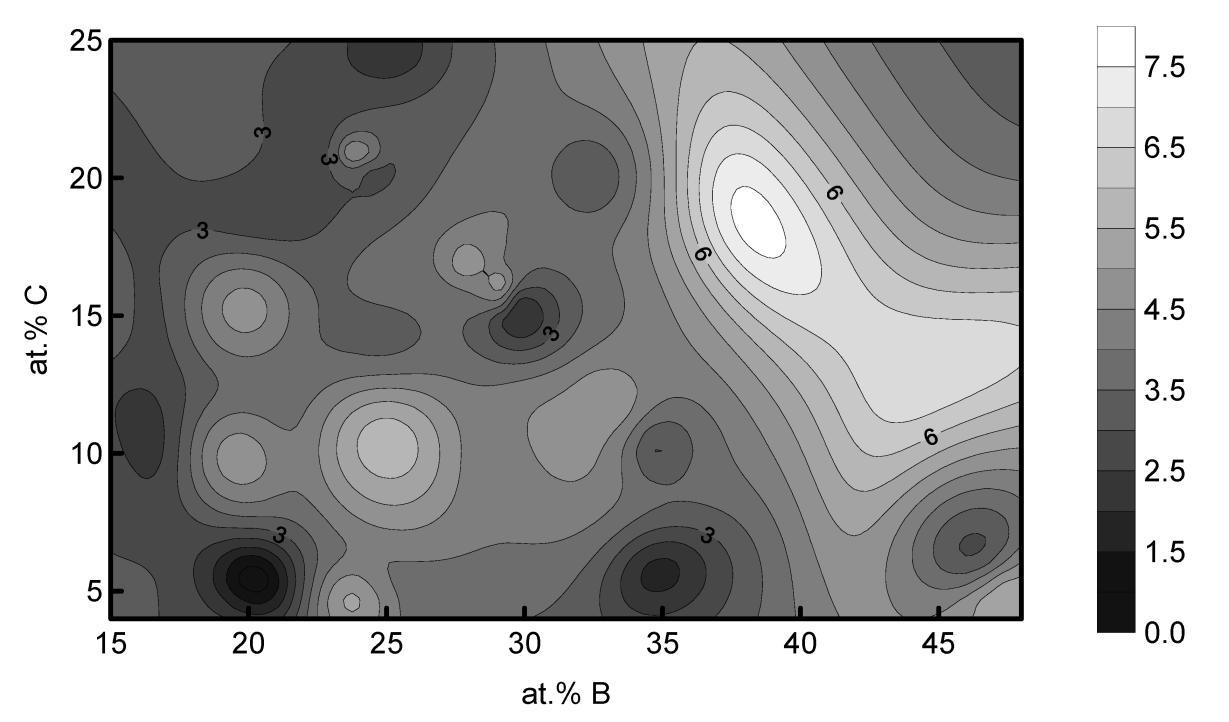
Fig. 2. The surface of the critical diameter (maximum diameter of an amorphous casting) constructed by smoothing an arbitrary data set of triple alloys (95 points from literary sources).
The atomic structure of glasses demonstrating the absence of long-range order in the arrangement of atoms ( Fig. 3) determine their properties, in particular mechanical. In terms of strength and specific strength, they significantly exceed the corresponding crystalline alloys due to the impossibility of using the mechanisms of accommodational deformation of a dislocation or twin type. The conditional yield stress of OMS reaches ~ 2 GPa for OMS based on Cu, Ti and Zr, ~ 3 GPa based on Ni, ~ 4 GPa based on Fe, ~ 5 GPa based on Fe and Co, and 6 GPa for cobalt alloys. The structure of metal glass also provides elastic deformation of up to 2%, which in combination with a high yield strength leads to large values of the stored energy of elastic deformation (indicators σy2 / E and σy2 / ρЕ, where σy, ρ and Е are the yield strength, density and Young's modulus, respectively). It should be noted that recent studies indicate the presence of atomic clusters in the OMC [9].
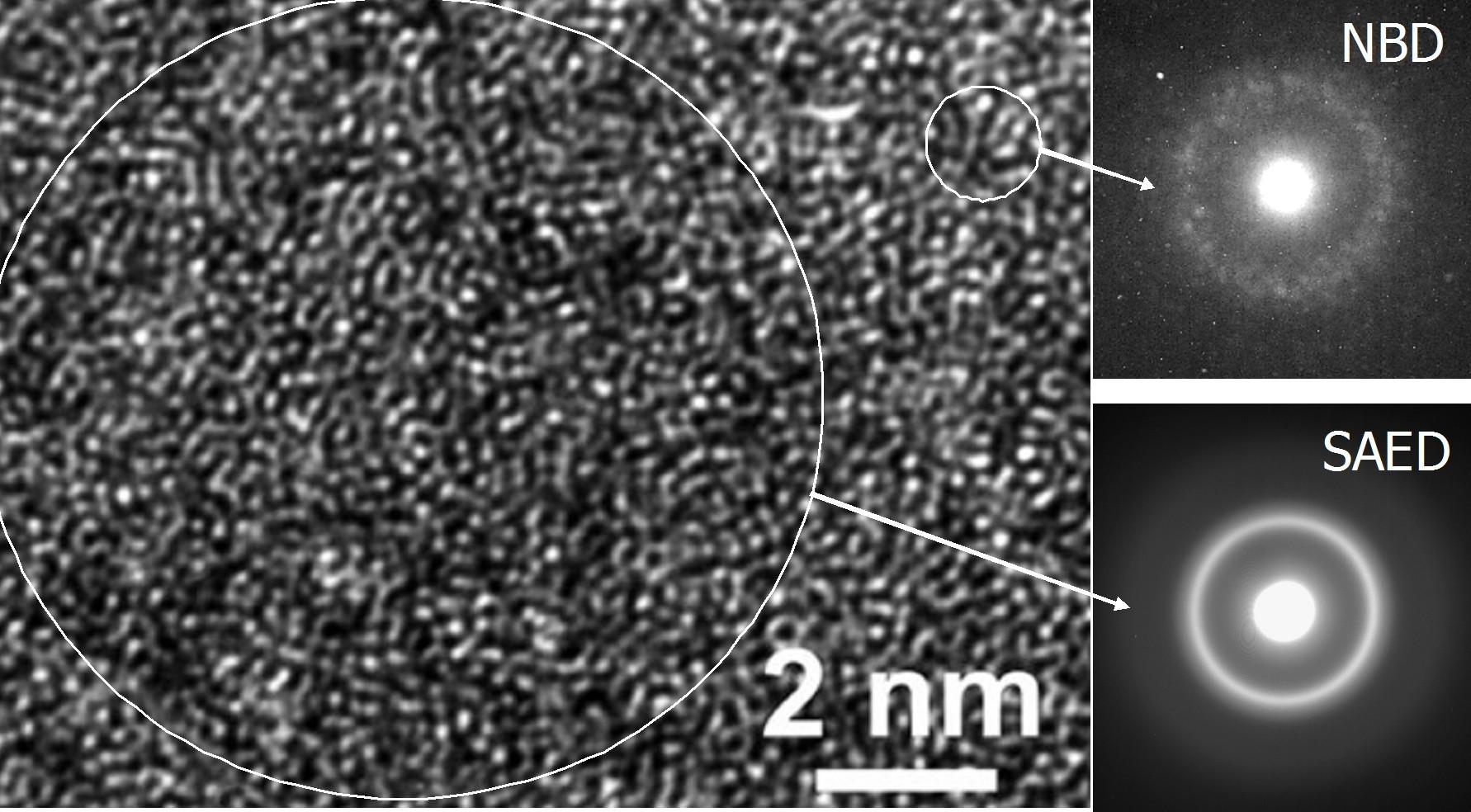
Fig. 3 . Image of high resolution transmission electron microscopy and diffraction patterns from a selected region of submicroscopic size (SAED) and nanoscale (NBD). The absence of long-range order in the arrangement of atoms is noticeable. The size of the scattering regions is shown conditionally in circles. (In Russia, the study of the structure of compulsory medical insurance is carried out, in particular, by A.S. Aronin and G.E. Abrosimov).
OMSs possess not only high strength, hardness, wear resistance and high values of elastic deformation before the onset of plastic deformation, but also high corrosion resistance, including spontaneous passivation in some solutions. High hardness, wear resistance, surface quality of the OMC, as well as fluidity during heating determines their use in micromachines as transmission mechanisms (gears), components of high-precision mechanical systems. OMSs based on iron and cobalt with saturation magnetization up to 1.5 T have record low values of coercive force less than 1 A / m and are actively used as soft magnetic materials. It should be noted that in Russia metal scientists based on iron and cobalt were engaged in such scientists as A.M. Glezer, S.D. Kaloshkin and many others.
The glass transition phenomenon observed during the transition from liquid to glass and devitrification upon heating is one of the most important problems of solid state physics that have not been completely resolved. Namely, are the amorphous and liquid phases the same phase, only observed at different temperatures, or is there a phase transition from the liquid state to the amorphous state and vice versa, and if so, what kind of phase transition is this? Some successes have been achieved using computer simulation, but there is still no complete clarity.
For a long time, it was not clear where such a property of “superfluid” metal comes from: “brittleness”: a strong deviation of the temperature dependence of its viscosity from the Arrhenius law, while the viscosity of an equilibrium liquid above the liquidus temperature (Tl) follows this law. The author and colleagues did not show in-situ X-ray diffraction by synchrotron radiation that the Pd42.5Cu30Ni7.5P20 alloy was actively formed in the atomic structure near the glass transition temperature of metal clusters covalently bound to P, which correlated with the temperature dependence of the viscosity of the liquid [10] upon cooling melt, as schematically shown in Fig. 4 . Note that the viscosity of a liquid when cooled to Tg changes by 10 orders of magnitude.
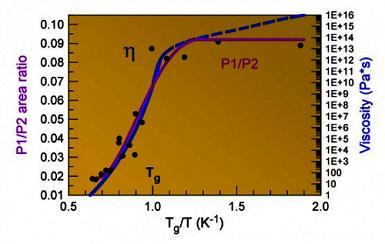
Fig. 4 . The ratio of the area under the peak of the radial distribution function (RDF) formed by Ni, Cu-P type atomic pairs to the peak area formed by other atomic pairs (P1 / P2) illustrates the rapid structural changes near Tg, and their absence below Tg and above Tl. On the right y-axis, viscosity is plotted on a logarithmic scale. The abscissa shows the return temperature normalized through Tg: equilibrium liquid below about 0.7 (small structural changes in temperature), supercooled liquid up to Tg / T = 1 (strong changes), and glass above.
The OMC perfectly and uniformly deform plastically when heated to a region of supercooled liquid before crystallization (above Tg, but below Tx — crystallization temperature of the supercooled liquid [11,12]), and can be used as models for dies ( Fig. 5 ).
Traditional methods of processing metals by pressure at a low cost of alloy components often require expensive processing steps of the source block to obtain the final product. This leads to a large amount of waste material. In the case of OMS, by means of rapid heating to the region of supercooled liquid, it is possible to obtain a product with high surface quality in one stage, as in superplastic molding. But, due to the absence of grain boundaries, OMS will be preferable for microobjects than superplastic alloys due to the extremely high surface quality.
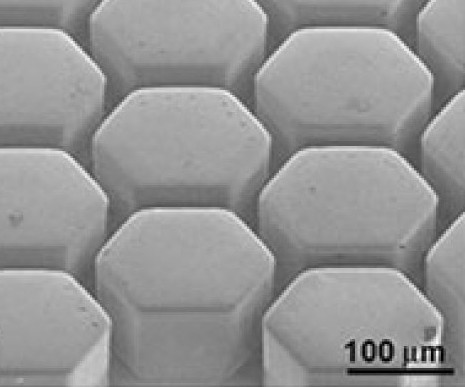
Fig. 5 . OMS surface after microforming in the area of supercooled liquid.
Nevertheless, one of the main obstacles to the wider use of glassy alloys is their limited ductility at room temperature [13]. Many OMS are destroyed before the start of macroplastic deformation during the formation of one shear band.
This is due to softening of the sample in local shear deformation bands (bands 10–20 nm thick, in which the material begins to flow under the action of high local stresses) and further localization of deformation in these bands, in contrast to crystalline alloys in which strain hardening leads to more uniform deformation in multiple slip bands. However, if several shear bands with alternating localization of deformation arise ( Fig. 6), then up to a certain point, macroscopically uniform deformation of the sample can occur, which is preferable for obtaining more plastic OMCs [14]. In this regard, the study of the process of nucleation and propagation of shear bands in the OMC is of great interest. As a result of improved compositions, record high fracture toughness values of the order of 100 MPa√m were obtained for the Zr61Ti2Cu25Al12 [15] and Pd79Ag3.5P6Si9.5Ge2 [16] OMCs, as well as alloys of hypereutectic compositions that are not embrittled during structural relaxation [17]. The recently proposed method of cyclic treatment in liquid nitrogen, due to the difference in the thermal expansion coefficients of atomic clusters with high and low densities, leads to the “rejuvenation” of metal glass (its transition to an increased energy state) and its plasticity [18].
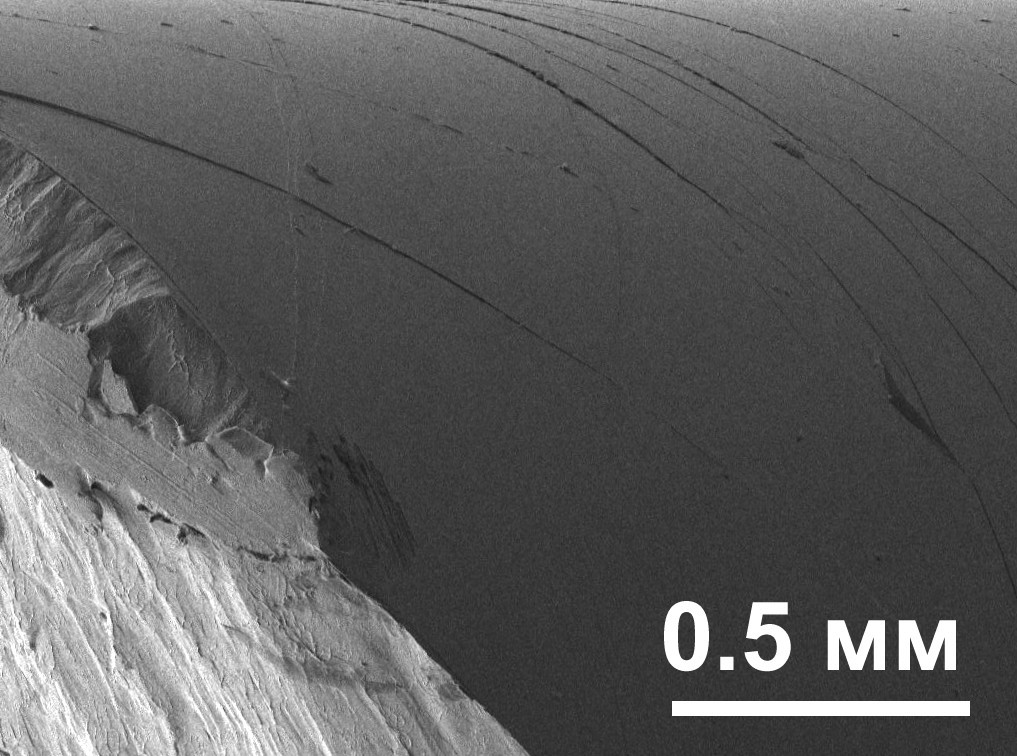
Fig. 6 . Shear bands on the lateral surface of the sample (top) after fracture. The destruction surface in the lower left corner.
Today, two-phase materials (composite type) are becoming more widespread, since they are able to combine, in an optimal ratio, the required set of operational properties. MHIs have uniquely high strengths, but, as a rule, lack of ductility, especially tensile. Crystalline alloys have high ductility, and polymeric materials also have low density. Therefore, the creation of a new class of light and durable two-phase materials based on metal glass and a crystal (or polymer) helps to solve the problem associated with improving mechanical structural materials, i.e. to obtain a material with a uniquely high rate of specific strength and ductility.Fig. 7 ).
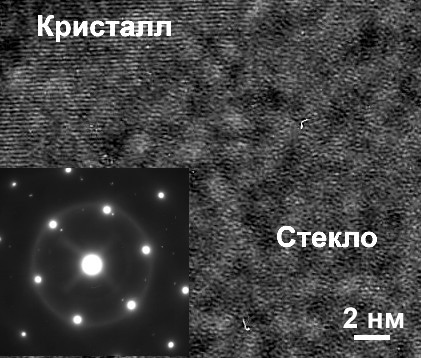
Fig. 7 . High resolution transmission electron microscopy image (two regions) and diffraction patterns from the selected region of submicroscopic size (bright spots are visible from the crystalline phase and halo from amorphous).
A promising material is nanostructured metal glasses ( Fig. 8 ) recently obtained in collaboration with the ICM Department , which can be used as materials for medicine (high corrosion resistance and good cell adhesion) and catalysis (developed surface) [21].
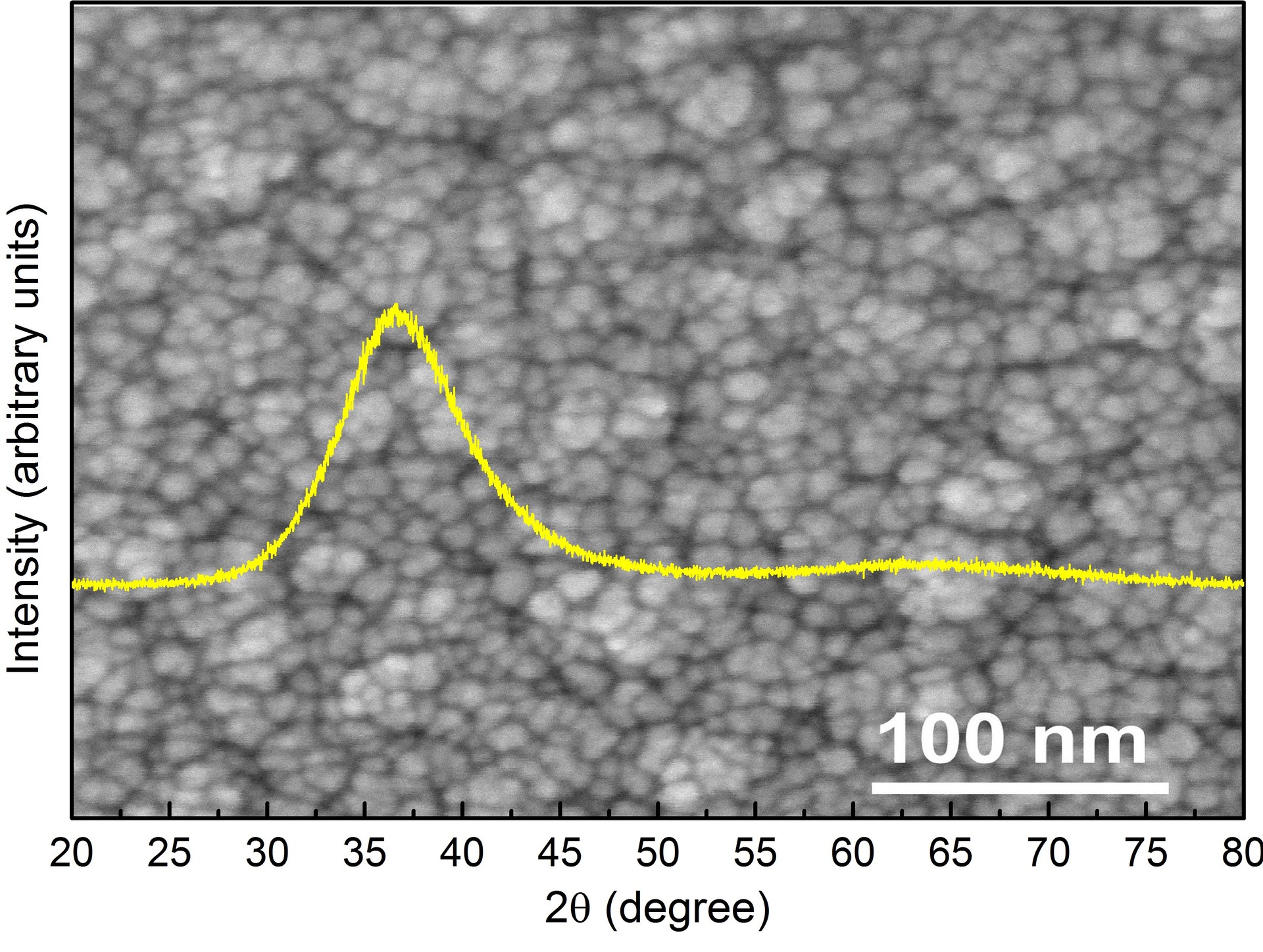
Fig. 8 . The surface of Zr-Pd nanostructured metal glass (SEM) as an insert in the X-ray spectrum obtained from the sample.
It has recently been shown that the homogeneous amorphous oxides formed on the surface of the Ni-Nb OMC have an amorphous structure without defects in the form of grain boundaries inherent in crystals, through which an electric current leak can pass. They demonstrate semiconductor properties, and their conductivity changes from electron to hole after annealing [22]. This material corresponds to a Schottky diode with a very low reverse current, the conductivity of which can be controlled by annealing in oxygen ( Fig. 9 ).

Fig. 9 . Volt-ampere characteristic of the natural amorphous oxide on the surface of the OMC Ni-Nb (blue curve) and oxide grown upon annealing at 300 C (red curve).
In conclusion, it should be noted that metal glasses after a half-century history are still of great interest for studying their unusual properties and structure, and biphasic glass-crystal materials are very promising for practical use as high-strength structural materials in areas where the cost is slightly higher material is not a big deal. Of course, one should not expect that OMC and two-phase materials, even when the compositions are cheaper, will replace structural steels or aluminum alloys in construction and heavy engineering. However, they, and two-phase materials such as glass-crystal, are already finding wider application in certain areas where they are superior to competitors: orthopedic screws in medicine (biocompatibility), micromachines (formability, wear resistance),
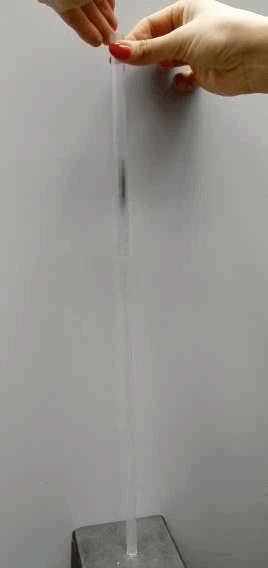
Fig. 10 This example illustrates the high ability of the OMC to store the energy of elastic deformation when the ingot falls from a certain height in the tube to the anvil.
Literary sources
1. www.boeing.com/commercial/787family/programfacts.html K. Lu, The Future of Metals, Science 328, 319 (2010).
2. W. Buckel, R. Hilsch Z. Phys. 138, (1954) 109-120.
3. YW Kim, HM Lin & TF Kelly Acta Metall. 37 (1989), 247–255.
4. W. Klement, RH Willens and P. Duwez Nature. 1987, (1967) 869.
5. HS Chen, Acta Metall. 22, (1974) 1505
6. HW Kui, AL Greer and D. Turnbull Appl. Phys. Lett. 45 (1982) 716.
7. DV Louzguine-Luzgin, A. Inoue, “Bulk Metallic Glasses. Formation, Structure, Properties, and Applications ”Handbook of Magnetic Materials, Edited by KHJ Buschow, Elsevier 21, 2013, 131-171.
8. DV Louzguine-Luzgin, DB Miracle, L. Louzguina-Luzgina, and A. Inoue, Journal of Applied Physics, 108, (2010) 103511.
9. AI Oreshkin, NS Maslova, VN Mantsevich, SI Oreshkin, SV Savinov, VI Panov, DV Louzguine-Luzgin, JETP Letters, 94, (2011) 58-62.
10. DV Louzguine-Luzgin, R. Belosludov, AR Yavari, K. Georgarakis, G. Vaughan, Y. Kawazoe, T. Egami and A. Inoue. J. Appl. Phys. 110, (2011) 043519.
11. G.E. Abrosimova, A.S. Aronin, I.I. Zverkova FMM, 94, (2002), 1-6.
12. SD Kaloshkin., IA Tomilin., Thermochimica Acta, 280/281, (1996), 303-317.
13. MF Ashby, AL Greer, Scripta Mater. 54 (2006) 321.
14. DV Louzguine-Luzgin, V. Yu Zadorozhnyy, N. Chen, SV Ketov, Journal of Non-Crystalline Solids. 396–397 (2014) 20–24.
15. Q. He, Y.-Q. Cheng, E. Ma, J. Xu, Acta Materialia 59, (2011), 202–215.
16. MD Demetriou, ME Launey, G. Garrett, PJ Schramm, DC Hofmann, WL Johnson, &, RO Ritchie, Nature Materials, 10, (2011) 123.
17. M. Aljerf, K. Georgarakis, AR Yavari, Acta Materialia, 59 (2011) 3817-3824.
18. SV Ketov, YH Sun, S. Nachum, Z. Lu, A. Checchi, AR Beraldin, HY Bai, WH Wang, DV Louzguine-Luzgin, MA Carpenter & AL Greer, Nature, 524, (2015) 200–203 .
19. SG Zaichenko, NS Perov, and AM Glezer, Journal of ASTM International, 7, (2010) 1102479.
20. AA Tsarkov, A. Yu. Churyumov, V. Yu. Zadorozhnyy and DV Louzguine-Luzgin, J. Alloys and Comp. in press.
21. SV Ketov, XT Shi, GQ Xie, R. Kumashiro, A. Yu. Churyumov, AI Bazlov, N. Chen, Y. Ishikawa, N. Asao, HK Wu and DV Louzguine-Luzgin, Scientific Reports, Vol. 5, (2015) 7799.
22. AS Trifonov, AV Lubenchenko, VI Polkin, AB Pavolotsky, SV Ketov and DV Louzguine-Luzgin, Journal of Applied Physics 117, (2015) 125704.
2. W. Buckel, R. Hilsch Z. Phys. 138, (1954) 109-120.
3. YW Kim, HM Lin & TF Kelly Acta Metall. 37 (1989), 247–255.
4. W. Klement, RH Willens and P. Duwez Nature. 1987, (1967) 869.
5. HS Chen, Acta Metall. 22, (1974) 1505
6. HW Kui, AL Greer and D. Turnbull Appl. Phys. Lett. 45 (1982) 716.
7. DV Louzguine-Luzgin, A. Inoue, “Bulk Metallic Glasses. Formation, Structure, Properties, and Applications ”Handbook of Magnetic Materials, Edited by KHJ Buschow, Elsevier 21, 2013, 131-171.
8. DV Louzguine-Luzgin, DB Miracle, L. Louzguina-Luzgina, and A. Inoue, Journal of Applied Physics, 108, (2010) 103511.
9. AI Oreshkin, NS Maslova, VN Mantsevich, SI Oreshkin, SV Savinov, VI Panov, DV Louzguine-Luzgin, JETP Letters, 94, (2011) 58-62.
10. DV Louzguine-Luzgin, R. Belosludov, AR Yavari, K. Georgarakis, G. Vaughan, Y. Kawazoe, T. Egami and A. Inoue. J. Appl. Phys. 110, (2011) 043519.
11. G.E. Abrosimova, A.S. Aronin, I.I. Zverkova FMM, 94, (2002), 1-6.
12. SD Kaloshkin., IA Tomilin., Thermochimica Acta, 280/281, (1996), 303-317.
13. MF Ashby, AL Greer, Scripta Mater. 54 (2006) 321.
14. DV Louzguine-Luzgin, V. Yu Zadorozhnyy, N. Chen, SV Ketov, Journal of Non-Crystalline Solids. 396–397 (2014) 20–24.
15. Q. He, Y.-Q. Cheng, E. Ma, J. Xu, Acta Materialia 59, (2011), 202–215.
16. MD Demetriou, ME Launey, G. Garrett, PJ Schramm, DC Hofmann, WL Johnson, &, RO Ritchie, Nature Materials, 10, (2011) 123.
17. M. Aljerf, K. Georgarakis, AR Yavari, Acta Materialia, 59 (2011) 3817-3824.
18. SV Ketov, YH Sun, S. Nachum, Z. Lu, A. Checchi, AR Beraldin, HY Bai, WH Wang, DV Louzguine-Luzgin, MA Carpenter & AL Greer, Nature, 524, (2015) 200–203 .
19. SG Zaichenko, NS Perov, and AM Glezer, Journal of ASTM International, 7, (2010) 1102479.
20. AA Tsarkov, A. Yu. Churyumov, V. Yu. Zadorozhnyy and DV Louzguine-Luzgin, J. Alloys and Comp. in press.
21. SV Ketov, XT Shi, GQ Xie, R. Kumashiro, A. Yu. Churyumov, AI Bazlov, N. Chen, Y. Ishikawa, N. Asao, HK Wu and DV Louzguine-Luzgin, Scientific Reports, Vol. 5, (2015) 7799.
22. AS Trifonov, AV Lubenchenko, VI Polkin, AB Pavolotsky, SV Ketov and DV Louzguine-Luzgin, Journal of Applied Physics 117, (2015) 125704.