Back to the future: practical proof of the Tomonaga-Luttinger theory after almost 56 years

Many technologies have changed dramatically since their invention. Their improvement was fueled by various studies and discoveries, each of which found new ways of implementation, be it materials, system models, or new algorithms. Visually, one of the most striking examples are computing devices. Once they occupied whole rooms and weighed several tons, but now each of us has a mobile phone, whose power is many times larger than those of dimensional computers. But the process of minimizing devices and their components is far from complete, for as long as there is room to reduce, scientists will invent new ways to achieve this. Today we will talk about the study, which can greatly influence the process of minimization, or more precisely, about the experimental confirmation of the theory of one-dimensional electrons, which is almost 56 years old. Go.
Background to research
In the distant 1950s, the Japanese physicist Shin-ichiro Tomonaga proposed a new theoretical model at the time that describes the interaction of electrons in a one-dimensional conductor. Further, in 1963, Joaquin Luttinger made some changes to the theory. The fact is that in the theory, under certain restrictions, second-order interaction between electrons can be described as bosonic interactions. Luttinger reinterpreted the theory, taking into account the Bloch waves. This showed that Tomonaga’s constraints are not needed to implement the model.

Shinichiro Tomonaga (1953)
In essence, this model is a description of the behavior of electrons with the help of two quasiparticles. They differ from each other in that the first has zero spin and charge, like an electron, and the second one has charge 0, but the spin is 1. At the same time, the quasi-particles move at different speeds. The theory also states that acting on a single charge or spin of an electron can cause the reaction of all electrons.
It is extremely difficult to test this theory experimentally, for scientists have so far failed to fully control the interaction of electrons. However, in this study, they found a way out of their predicament by applying cold atoms.
More than lucidly the essence of cold atoms reveals Alexei Akimov in the video above.
In this study, a Fermi gas model of fermionic 6 Li was created, since its repulsive s-wave interactions are easily manipulated. In order to change the required parameter — the dynamic structure factor S (q, ω) of density fluctuations (“charge”) —Bragg spectroscopy was used.
The researchers do not deny that this method of measurement has already been used previously, but their method has several important features. First, the atoms were “caught” in an optical trap consisting of three mutually orthogonal infrared laser beams, each of which passed through a retro reflector *, while the polarization of each reflected beam was rotated 90 ° in order to form a trap without a lattice.
Retroreflector * - a device to reflect the beam back to its source with minimal dispersion.Thereafter, it was measured the number of atoms - 1.4h10 5 , and also their temperature - 0.05 T F . In this particular case, T F is the Fermi temperature of each spin state, taking into account that there are no interactions.
Example of retroreflector
Further, the depth of the trap was increased, and the polarization of the reflected rays was rotated so as to form a three-dimensional lattice with a depth of VL = 7 E r , where
E r = h 2 / (2mλ 2 ) is the recoil energy;
h is Planck's constant *;
m is the atomic mass
λ = 1.064 nm is the wavelength of light.
In order to achieve the desired grating depth (2.5 E r), the scattering length was corrected. To compensate for the limiting envelope of infrared rays, an unreflected 532 nm beam was applied along each axis, which was subjected to a blue detuning * .
Laser detuning * - tuning the beam to a frequency different from the resonance of the quantum system. Setting the laser to a frequency above the resonance is called blue ( blue-detuning ).Subsequently, the compensating beam was gradually turned off, as was the vertical infrared beam. In parallel, the intensity of the two remaining for the formation of an already two-dimensional lattice (15 E r ) increased . Due to this, the two-dimensional lattice created a bundle of practically isolated one-dimensional tubes. They can be described using two parameters: axial harmonic oscillation - ωz = (2π) 1.3 kHz and radial harmonic oscillation - ω⊥ = (2π) 198 kHz.
Conducting these manipulations led to a decrease in the total number of atoms in the experiment to N = 1.1x10 5 .
In Bragg spectroscopy involved two laser beams with vectors k 1 and k 2, as well as the frequency difference ω. The rays pass at an angle θ relative to each other and intersect the atoms symmetrically with respect to the line perpendicular to the tube axis (z). These two beams lead to a stimulated two-photon transition, which provokes the "imposition" of the ground state of the quantum system on the frequency excitation ω and the z-component of the pulse q = | k 1 - k 2 | = 2k sin (θ / 2), where k = | k 1 | = | k 2 |.
The angle between the rays was set to θ / 2 ~ 4.5 °, which leads to q / k F ≃ 0.2 for the central tube with the number of atoms N m = 60.
As it has already become clear, the angle between the laser beams determines the q value, which should be lower than Fermi pulse. More on this later.
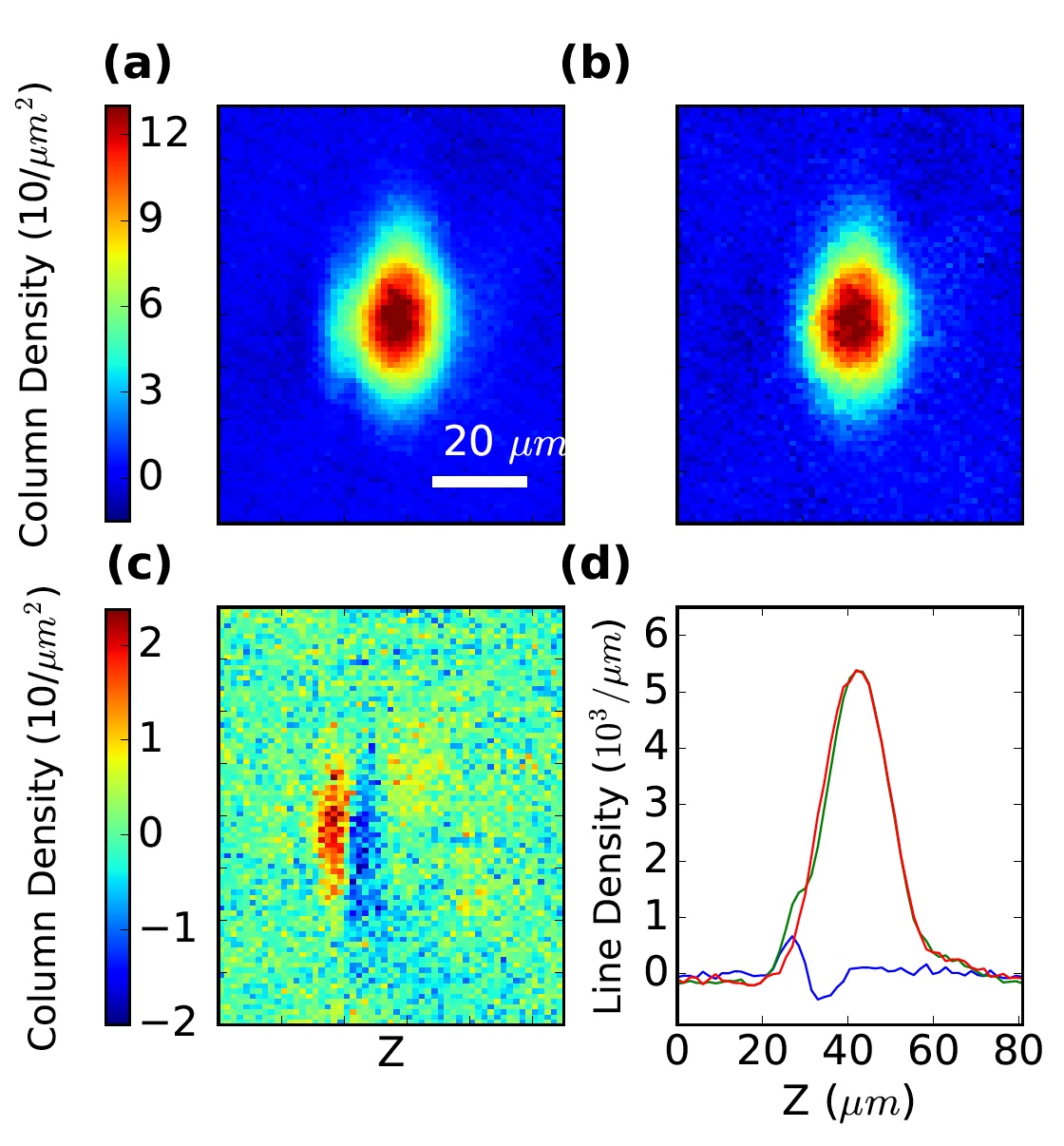
Image No. 1
Bragg rays work for 300 microseconds, which is about 2 times less than the axial period, but more compared to ω -1 . An important nuance, since this simplifies the analysis and reduces the pulse-temporal broadening.
As soon as the Bragg beam is applied to the test sample, the optical trap beams are switched off. After 150 microseconds, images are obtained using phase-contrast microscopy. The experiment is repeated, but without the influence of the Bragg rays, to obtain a "reference" image.
The image a and b shows the column density of the experimental version and the "reference", respectively. On c - the difference between them. d- a graph of the ratio of all three previous indicators: a, b and c.
Researchers point out that the Bragg signal was in the linear response mode due to changes in the intensity of its beam caused by a change in the duration of exposure. In this mode, the frequency of stimulated Bragg transitions quadratically depends on the intensity of the laser radiation.

Image No. 2
As can be seen from the graph above, when the radiation intensity is less than 55 mW / cm 2 , the pulse transmission is in the linear response mode over the entire range of interaction force available in the experiment.

Image number 3
The graph above shows the ratio of the Bragg signal and frequency, where each point corresponds to 20-30 experimental attempts for each value of ω and constant q.

Image №4
The graph above shows the results of measuring the value of ω. As the interaction force increases to 400a 0 , the frequency value also increases. With further increase, over 400, heating and loss of atoms was observed, which is most likely due to three-component recombination due to the unstable upper branch during the transition from a three-dimensional to a two-dimensional lattice.
For further measurements, it was decided to calculate the structural factor at a temperature of 200 nK and compare these results with previous ones in the experiment. In such a calculation, the only parameter that can be manipulated is excitation scaling. Peak excitation values are shown in graph 4 as red dots. But the dotted line shows the theoretical results. It is obvious that the experimental theoretical results almost coincide. These results are the first evidence of an experimental demonstration of changes in the rate of collective excitation in a one-dimensional Fermi gas in response to the interaction.
To get acquainted with the details of this study I strongly recommend to look here (the report of scientists) .
Epilogue
Scientists were able to successfully measure the dynamic response inside a one-dimensional two-component fermionic system through the use of Bragg spectroscopy. This experiment was able in practice to confirm the veracity of the Tomonag-Luttinger theory.
Scientists believe that the ability to manipulate the power of interaction through Feshbach resonance will open the door to future research that can cross the boundaries described in the Tomonaga-Luttinger theory.
This work is extremely difficult to call easy, because it is fraught with many problems in conducting the experiment and in the very measurements of its results. However, the desire to learn something new, as well as the importance of something new for the development of the Earth's technosphere, is immeasurably great. Familiarizing yourself with such studies, you understand how complex the world around us is. For centuries, we have been trying to understand, simplify and subordinate it, but with each new discovery a lot of new questions appear that complicate what we have supposedly simplified.
Thank you for staying with us. Do you like our articles? Want to see more interesting materials? Support us by placing an order or recommending to your friends, a 30% discount for Habr users for a unique analogue of the entry-level servers that we invented for you:The whole truth about VPS (KVM) E5-2650 v4 (6 Cores) 10GB DDR4 240GB SSD 1Gbps from $ 20 or how to share the server? (Options are available with RAID1 and RAID10, up to 24 cores and up to 40GB DDR4).
VPS (KVM) E5-2650 v4 (6 Cores) 10GB DDR4 240GB SSD 1Gbps until December for free if you pay for a period of six months, you can order here .
Dell R730xd 2 times cheaper? Only we have 2 x Intel Dodeca-Core Xeon E5-2650v4 128GB DDR4 6x480GB SSD 1Gbps 100 TV from $ 249 in the Netherlands and the USA! Read about How to build an infrastructure building. class c using servers Dell R730xd E5-2650 v4 worth 9000 euros for a penny?