Ask Ethan # 60: Why does energy disappear from the universe?
- Transfer
Background cosmic radiation in the universe once roasted everything around, but now it is almost close to absolute zero. Where did all the energy go?
I think that the coolest thing you can do is to disappear for a while, because it will give you a chance to reappear.
- Josh Michael Homme
If you think about it, the Big Bang is one of the most complex abstractions you can imagine. Of course, now the Universe is expanding, which means that before everything was closer to each other and the Universe was denser. But it was also hotter, so the particles in it were more energetic than today, when they are already more “cold”. This week, the question is won by Barry Purdo, who asks:
As I understand it, the cosmic microwave background radiation (CMI) is gradually cooled with the expansion of the Universe, and that the CMFI particles with red shift move to increasing wavelengths and decreasing energy levels. But where does the energy of these particles go?
Let's see and understand why this question is so deep.

It is easy to understand how the density falls as the Universe expands, and how - if it suddenly begins to shrink - its density will begin to grow. Indeed, density is only the amount of a substance in a certain region of space: mass density is mass per volume, quantitative density is quantity per volume, and energy density is energy per volume.
In the case of matter — atoms, gas, planets, stars, galaxies, even dark matter — you can intuitively associate this with space-time, which changes over time. If space-time expands, density decreases, if it contracts, it grows.
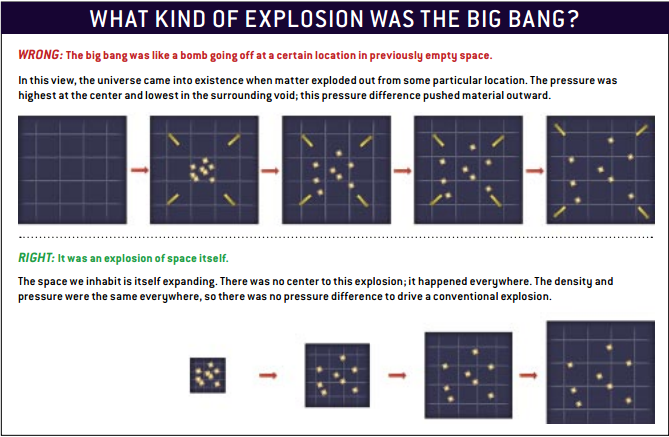
It is wrong to think that the Big Bang is like a bomb that exploded in empty space, where the pressure at the center of the explosion was great, and outside it was small.
In fact, the whole space exploded, the explosion did not have a center, and the density and pressure were the same everywhere. Therefore, the pressure difference that would lead to an explosion simply did not exist.
This is all due to a change in volume. The mass remains the same, the number of particles remains the same as the amount of energy. In an expanding universe filled with matter, the density changes as the universe expands.
But in the Universe filled with radiation - photons or particles of light in our case - a change in the volume of the Universe leads to some unexpected consequences.
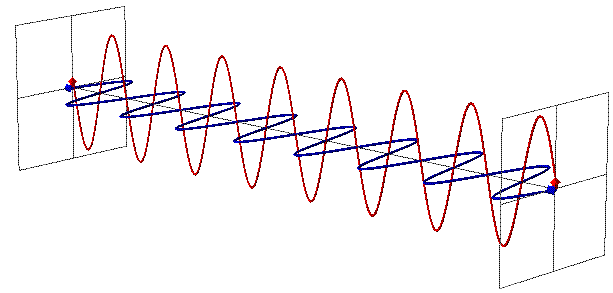
You are used to counting particles as particles, points in space. You consider them entities without size, so when the Universe expands (or contracts, although it does not), the particles remain the same. But photons do not behave like this.
A photon is not only a particle (although it is capable of colliding and interacting in the manner of particles), but it also behaves like an electromagnetic wave. And one of the most important fundamental characteristics of a wave is its length, which in the case of a photon determines its energy.

The longer the wave, the less energy, and the shorter the wave - the greater the energy. Now, in today's Universe size, a typical photon remaining from the early stages of the Universe development has energy corresponding to a temperature of 2.725 K. It can be converted into a wavelength using a set of constants - Boltzmann, Planck and the speed of light. Then we will find that the wavelength will be equal to 5.28 mm, that is, approximately equal to the length of the white part of the nail, when it is time to cut it.
About 189 of such wavelengths will fit in the meter. But in the past, due to the expansion of the universe, every meter of intergalactic space was less!
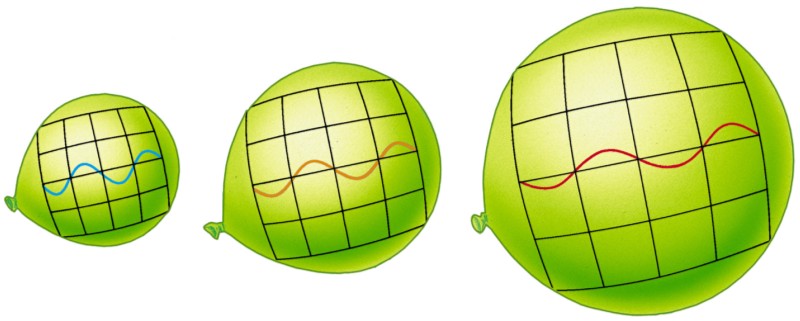
But this does not mean that fewer waves would fit in the same space. Quantitative density per unit volume remains constant. So what happens then? You could fit 189 waves of this light at a distance that has expanded to today's meter!
- When was the universe half the size of today? 189 waves in half a meter, or a wavelength of 2.64 mm
- When was the Universe sized 10% of today's size? 189 waves in decimeter, or a wavelength of 528 microns
- When was the Universe about 0.01% in size? 189 waves in one tenth of a millimeter, or a wavelength of 528 nanometers - visible light! (yellow green)
The further we go back - in times when the Universe was smaller - the more energy was contained in the radiation. The radiation observed today after the Big Bang came to us from the time when the first neutral atoms were formed: the cosmic surface of the last scattering.
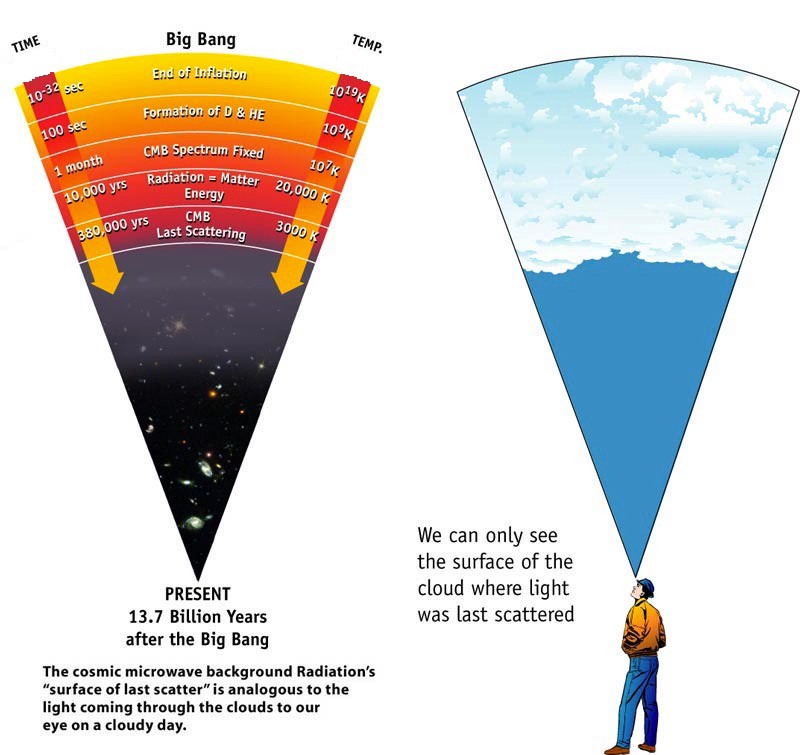
KMFI, the surface of the last scattering - the equivalent of light passing through the clouds and entering our eyes. We can only see the surface of the clouds, from which the light was reflected for the last time.
And this explains why there was a time when there were no neutral atoms (where the CMBR was emitted), when there were no atomic nuclei (since they were broken into pieces - and right after that the moment came when the lightest elements of the Universe were synthesized), when the protons and neutrons were broken up into quark-gluon plasma, and even earlier, when everything was so hot that matter-antimatter pairs were spontaneously created from incredibly high-energy gamma rays that flooded the Universe.
It also explains why the residual radiation looks so much shifted into the microwave part of the spectrum. These are simple predictions arising from the physical laws and concepts of the Big Bang.
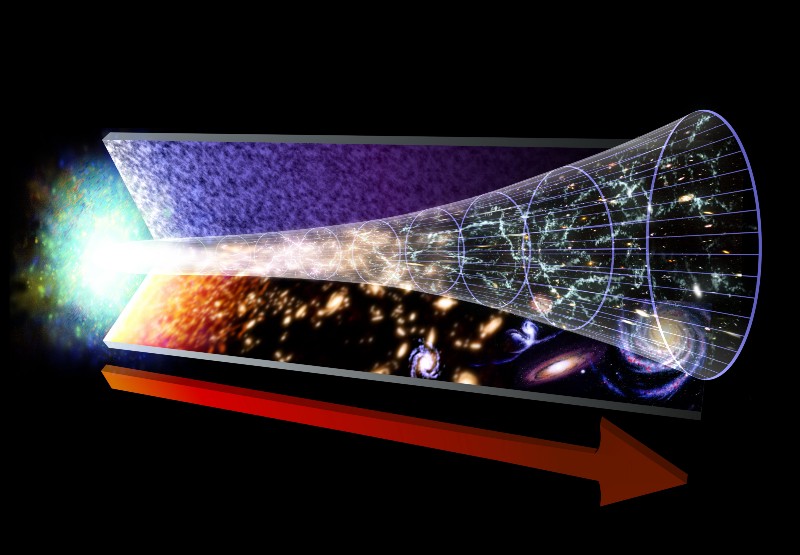
But this may cause you the same questions as Barry. Is energy not conserved? And if today its level is low, does it mean that it is lost, and therefore not preserved? (Strictly speaking, in the GTR there is no definition of energy - but we will not use it in order to avoid the answer).
The radiation energy is not lost; let's look at the analogy. Imagine that you have a ball that you have inflated and tied up, and in an inflated state it is in balance with the environment. You can measure the amount of energy in the air inside the ball and be satisfied with it.
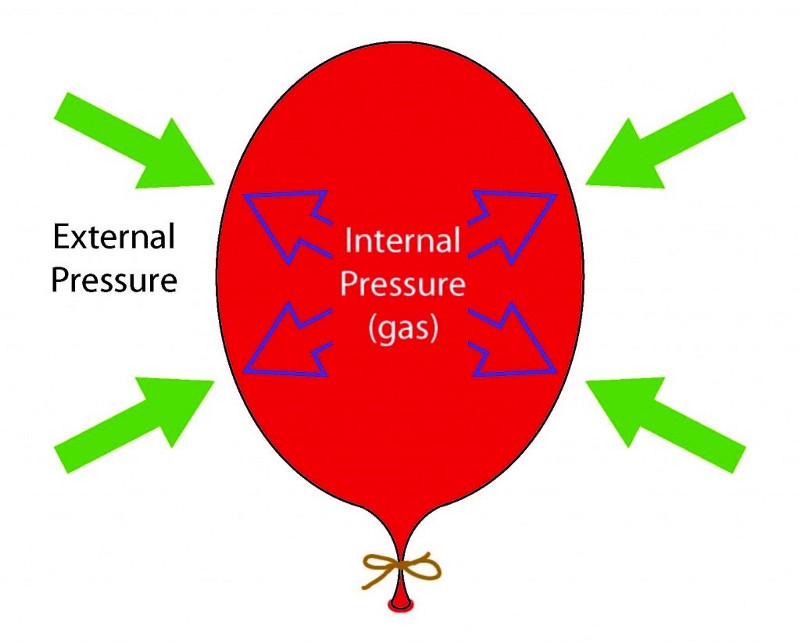
Now we will deal with it rigidly, and we will lower it into liquid nitrogen at a temperature of 77 K. Nitrogen will suck the heat from the air molecules (and the ball), and the volume of the ball will fall.
But that is not all. Another principle works here: the molecules put pressure on the walls of the ball, which did not allow it to collapse inwards, and when they lost energy, the force exerted by them was not enough, and the ball began to contract. If you pull the ball out of nitrogen, and let the air heat it, it will gain energy and inflate the ball again, pushing the walls from the inside.
The idea of applying force in a certain direction, when something moves in it, or in the opposite direction, describes the physical concept of work. If you push it out and something moves inwards, you do negative work by taking energy from the system. If you push it out and it moves out, you do a positive job adding energy to the system. That's what a balloon is like - perhaps the simplest example of this combination of power, distance and work.

In the case of the Universe, the photons work like the air inside the ball: they press outward while the Universe expands and perform positive work. The photons lose their energy, but the energy passes into the Universe itself, and in a reversible way! In other words, if the Universe shrinks or even re-collapses, the energy added to it by photons will return to them.
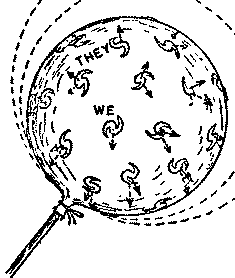
So, where does the photon energy go in the expanding universe? The photon energy does the work, transferring it to the Universe itself.
Thank you for the wonderful question, Barry, and I hope that the explanation has been made clear for you and for the rest. Send me your questions and suggestions for the following articles.