Stable high voltage power supply for PMT
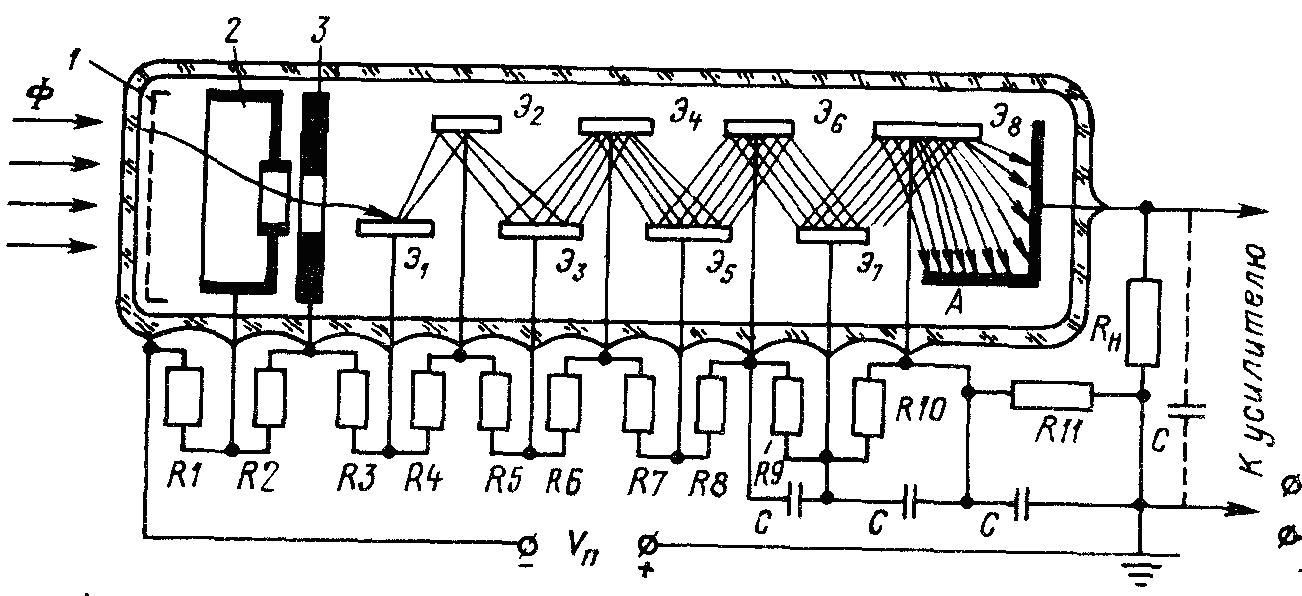
The use of a photoelectronic multiplier is a very simple way to obtain the highest sensitivity of a photodetector, up to registering single photons with excellent speed. And given the mass of PMTs produced in the USSR and still lying in warehouses, it is also relatively inexpensive (modern “proprietary” PMTs are still indecently expensive for amateur use). But for supplying a photomultiplier, a voltage source of 1-3 kilovolts is needed, and moreover, it is very stable.
The fact is that the sensitivity of the PMT depends on the anode voltage exponentially and very sharply: it increases 10 times with an increase in voltage by 80-300 V, depending on the type of PMT. And if it is necessary to ensure stability of gain at a percentage level, for some PMTs it is necessary that the voltage does not change by more than 0.1-0.3 V!
In this article, I give a diagram of a high voltage source for a PMT, which has proven itself in the laboratory. It provides an output voltage of several hundred to 1500 V with an output current of up to 1 mA and stability of no worse than 0.2 V per hour with a constant current consumption after heating. A simple alteration increases the upper voltage limit to 3 kV, however, at the cost of less stability.
Scheme

The basis of the source is a push-pull inverter operating on a transformer for CCFL lamps. The inverter is made on the basis of a domestic chip for electronic ballasts - KF1211EU1. I could not find any equal on this chip on sale: it can directly control the gates of field-effect transistors and for operation it needs only two external elements (a timing resistor and a capacitor), while it works regularly from 5 V and is inexpensive. Unfortunately, NPO Delta has not been producing this chip for a long time, but it is still on sale and it is not difficult to get it. This microcircuit has no means of regulating the duty cycle, but we don’t need it - the output voltage is controlled by changing the supply voltage of the inverter output stage. A key element is the VT1 dual n-MOS transistor type IRF7341.
The inverter operates at a frequency of 40 kHz. It has been experimentally established that at this frequency the transformer used works best and has the best efficiency. This frequency is set by the chain R1C1.
I used the transformer from the TMS91429CT series, which has two identical primary and two identical secondary windings isolated from each other. This makes it possible to exclude the voltage multiplier with large losses, replacing it with two single-cycle rectifiers, the output voltages of which add up, forming a not quite ordinary-looking, but essentially the same two-stroke rectifier. The configuration described in the diagram works with this transformer somewhat better than the classic "with a tap from the middle." If higher voltages are needed, a doubler can be assembled in each of the “halves”.
Resistor R8 and capacitor C9 form a filter that reduces high voltage ripple. Resistor R10 reduces the risk of fatal electric shock: despite the fact that the direct current generated by this source does not pose any serious danger, the energy stored in capacitor C9 is sufficient to kill, and the peak current of its discharge is limited to ~ 60 mA at maximum voltage reduces this possibility (with short-term - hundredths of a second - exposure, this current is usually not fatal). However, at a current of 1 mA, 22 V drops on this resistor, which is most likely unacceptable. Therefore, if currents of more than a hundred microamps are needed, it will have to be removed, but in this case, remember that the output voltage of the source isdeadly . With the resistor R10, however, too, but the danger is not so high.
The output voltage, divided by a divider R7R9 500 times, is fed to the input of the error amplifier on the op amp DA1.2. A reference voltage is supplied to its second input (via a repeater on DA1.1), which sets the output voltage, which, in accordance with the division ratio of the R7R9 divider, will be 500 times higher (for example, at a reference voltage of 3 V, the output will be 1.5 kV). The gain of the error amplifier is selected experimentally. Its increase increases the accuracy of stabilization, but reduces stability. Capacitor C8 compensates for the delay in the feedback loop and ensures regulation stability. The ratio of the gain of the error amplifier and the time constant of the R6C8 circuit is a compromise between the accuracy of maintaining the output voltage and the time it takes to establish it.
The output voltage of the error amplifier is supplied to the control element - p-MOS transistor VT2. The transistor is completely closed when the voltage at the output of DA1.2 is close to the supply voltage (that is, if the high voltage is much higher than the set value), and fully opens when it is reduced to zero (at a very low voltage), which ensures its maintenance at a slightly higher level reference voltage multiplied by the division factor. Not all MOS transistors work well in linear mode, and the one indicated on the circuit makes this quite acceptable. The resistor R4 prevents the instability of the op-amp when operating on a capacitive load, which is the gate of the transistor.
A multi-turn potentiometer powered by a stabilized voltage source can be used as a reference voltage source, but with increased stability requirements it may not be enough, since even the best of such variable resistors “noise” to some extent, randomly changing the resistance small limits, even if the adjustment knob is not touched. To increase it, it is desirable to limit the range of smooth tuning to 100-200 V and introduce a switch for a discrete coarse voltage setting. Another option is to make a digital ION based on some kind of DAC.
This circuit gives a high voltage of a positive sign. It is convenient to use a negative supply voltage with a grounded anode to power the PMT. For this, the circuit will have to be adjusted - firstly, by changing the polarity of the diodes in the high-voltage part. Secondly, it is necessary to introduce another operational amplifier into the circuit. Instead of the R9R7 divider, we have an inverting amplifier with a gain of minus 1/500 on the DA2 op amp, and the resistors R9 and R7 are in its OOS circuit.
To get 3 kilovolts, you will have to replace the rectifiers in the secondary circuits with voltage doublers and increase R9 to 100 MΩ. At the same time, stability will worsen about the same two times.
Components and Installation
Capacitors and resistors of size 0805 or even 0603 can be used in low-voltage and low-current circuits. Capacitor C2 is tantalum. The capacitor C4 is a film capacitor, since a noticeable pulsed current flows through it and the ceramic SMD capacitor will warm up here and will quickly fail.
From the high voltage side, it is necessary to mount all AC circuits as short as possible, as otherwise they radiate strongly (however, remember to observe the insulation gaps). Diodes are dialed each of two series-connected 1000 V diodes. Due to the lack of fast 1000 V diodes in stores, SMD-version uses HER1008 output diodes, installed in two in series. To reduce the length of the terminals, they are bent under the diode housing and cut off, and thus, the diode is redone in SMD. In this case, the anode of one diode in pair is soldered to the cathode of the second directly and as close as possible to the output of the output from the housing, and not through the printed conductor. Capacitors C6 and C7 are also composed of four capacitors of 0.015 μF x 1000 V, size 1812, connected in series-parallel and soldered by a “whatnot” on each other. Capacitor C9 of arbitrary type - I used a battery from domestic K15-4, filled with a compound for reliability.

Resistor R8 - size 2512. R10 is composed of ten such resistors connected in series on a separate small board and filled with an insulating compound. You can do the same with R9, or use a resistor of the FHV-100 series. And it’s absolutely ideal to put a Caddock THV10 series divider. The voltage drift depends on the thermal stability of this resistor (and it is heated by the current passing through it). Thermal insulation of it, increasing the time to establish a stable voltage, however, sharply reduces its chaotic fluctuations, therefore ,recommended. Also, during installation, you should pay attention to possible leakage paths, which will also sharply reduce stability. On the printed circuit board, slots and windows should be provided that separate the high-voltage circuits from the low-voltage ones and between closely spaced conductors with sharply different potentials. And do not spare the alcohol - the slightest moisture, traces of rosin or little fingers - and the tension will gallop like a wild mustang. It goes without saying that the entire high-voltage part must be flooded with a compound, since otherwise the gaps would have to be made very large. And the large gaps are the large length of the conductors and strong radiation. During the work of the initial layout, where I used K78-1 capacitors, output diodes with slightly shortened leads and gaps, recommended for printed installation in air - at idle, the circuit consumed almost 200 mA at 1500 V, and the neon burned 10 cm from the structure. It was impossible to even look at the voltage shape on the primary windings of the transformer - a hundred volt span was induced on the oscilloscope probe. There could be no talk of any practical use of such a strongly emitting interference circuit. After the transition to SMD and the most compact installation (which required pouring - everything immediately breaks through in the air), the current consumed at idle dropped to a couple of tens of milliamps, and the neon bulb burned only close to the transformer winding. Of course, the finished device must be placed in a metal case equipped with a good high-voltage connector (for example, of the LEMO type). It was impossible to even look at the voltage shape on the primary windings of the transformer - a hundred volt span was induced on the oscilloscope probe. There could be no talk of any practical use of such a strongly emitting interference circuit. After the transition to SMD and the most compact installation (which required pouring - everything immediately breaks through in the air), the current consumed at idle dropped to a couple of tens of milliamps, and the neon bulb burned only close to the transformer winding. Of course, the finished device must be placed in a metal case equipped with a good high-voltage connector (for example, of the LEMO type). It was impossible to even look at the voltage shape on the primary windings of the transformer - a hundred volt span was induced on the oscilloscope probe. There could be no talk of any practical use of such a strongly emitting interference circuit. After the transition to SMD and the most compact installation (which required pouring - everything immediately breaks through in the air), the current consumed at idle dropped to a couple of tens of milliamps, and the neon bulb burned only close to the transformer winding. Of course, the finished device must be placed in a metal case equipped with a good high-voltage connector (for example, of the LEMO type). After the transition to SMD and the most compact installation (which required pouring - everything immediately breaks through in the air), the current consumed at idle dropped to a couple of tens of milliamps, and the neon bulb burned only close to the transformer winding. Of course, the finished device must be placed in a metal case equipped with a good high-voltage connector (for example, of the LEMO type). After the transition to SMD and the most compact installation (which required pouring - everything immediately breaks through in the air), the current consumed at idle dropped to a couple of tens of milliamps, and the neon bulb burned only close to the transformer winding. Of course, the finished device must be placed in a metal case equipped with a good high-voltage connector (for example, of the LEMO type).
PCB layout (I don’t give mine because it turned out to be not very successful and in the final design it was covered, like mold, by pockets of wall mounting, correcting the errors of the original design) should be done taking into account the fact that VT2 is heated and removes heat through the terminals (dissipated power can reach 2 watts). VT1 remains almost cold during operation. Also, pay attention to the land, especially in the vicinity of key transistors. The latter, along with DD1, is conveniently placed under the belly of the transformer, around which the ground can be separated by a gap, connecting it with the rest of the earth at a single point near the power connector.
And about the replacements. The transformer can be replaced by almost any similar transformer with the same winding configuration (i.e. two identical primary windings and two separate high-voltage windings) and the same overall power, and it may be necessary to select the switching frequency and capacitance of the capacitor C4. The transistor assembly VT1 can be replaced by similar separate n-MOS transistors with a voltage of source-drain of at least 20 V and a drain current of at least 3 A, capable of operating with 5 V on a gate. VT2 replacement is undesirable.
A bit about security
As I said, this device is deadly for life . Despite the fact that a current of several milliamps provided by this device is not dangerous even when passing along the “language-arm” path, the discharge of the capacitance at the output, although not guaranteed to kill, can very well do this, since the current reaches several amperes (!), and the discharge energy at maximum voltage is about 0.1 J, which is quite enough to cause ventricular fibrillation in the vulnerable phase. So be careful - especially during the setup process. At this time, I recommend replacing the capacitor C9 with a less capacious one.