
Transistor story: wading to the touch in the dark
- Transfer

Other articles in the series:
- Relay history
- The history of electronic computers
- Transistor history
- Internet history
The road to solid state switches was long and difficult. It began with the discovery that certain materials behave strangely in the presence of electricity - not in the way that theories that existed then predicted. This was followed by a story about how, in the 20th century, technology became an increasingly scientific and institutional discipline. Amateurs, novices and professional inventors, with virtually no scientific education, made serious contributions to the development of telegraph, telephony and radio. But, as we will see, almost all the advances in the history of solid-state electronics have occurred thanks to scientists studying at universities (and usually having a Ph.D. in physics) and working at universities or corporate research laboratories.
Anyone with access to a workshop and with basic skills in working with materials can assemble relays from wires, metal and wood. To create electronic lamps, more specialized tools are required that can create a glass flask and pump air out of it. Solid-state devices disappeared into a rabbit hole, from which the digital switch never returned, and plunged deeper into worlds that were understandable only to abstract mathematics and accessible only with insanely expensive equipment.
Galena
In 1874, Ferdinand Brown , a 24-year-old physicist from the School of St. Thomas in Leipzig, published the first of many important scientific works in his long career. The work “On the passage of electric currents through metal sulfides” was accepted in the journal Pogendorff's Annalen, a prestigious journal devoted to the physical sciences. Despite the boring headline, Brown's work described several surprising and cryptic experimental results.

Ferdinand Brown
Brown was intrigued by sulfides - mineral crystals composed of sulfur compounds with metals - thanks to the work of Johann Wilhelm Gittorf. Back in 1833, Michael Faraday noted that the conductivity of silver sulfide increases with temperature, which is completely opposite to the behavior of metal conductors. Hittorf compiled a thorough quantitative report on the measurements of this effect in the 1850s, for sulfides of both silver and copper. Brown, using a sophisticated experimental setup that pressed a metal wire to a sulfide crystal with a spring to ensure good contact, found something even stranger. The conductivity of the crystals depended on the direction — for example, the current could flow well in one direction, but when the polarity of the battery was reversed, the current could suddenly drop sharply. Crystals in one direction worked more like conductors (like normal metals), and in the other they worked more like insulators (like glass or rubber). This property has become known
At about the same time, researchers discovered other strange properties of materials such as selenium, which could be smelted from certain sulfide metal ores. Under the influence of light, selenium increased the conductivity and even began to generate electricity, and it could also be used for rectification. Was there any connection with sulfide crystals? Without theoretical models capable of explaining what was happening, confusion reigned in this area.
However, the lack of theory did not stop attempts to put the results into practice. In the late 1890s, Brown became a professor at the University of Strasbourg - recently annexed to France during the Franco-Prussian Warand renamed Kaiser Wilhelm University. There he was sucked into an exciting new world of radio telegraphy. He agreed with the proposal of a group of entrepreneurs to jointly create a wireless communication system based on the transmission of radio waves through water. However, he and his accomplices soon abandoned the original idea in favor of airborne signaling, which Marconi and others used.
Among the aspects of the radio that Brown's group sought to improve was the then standard receiver, coherer. It was based on the fact that radio waves forced metal filings to gather in a lump, which allowed the current from the battery to pass to the signal device. This worked, but the system responded only to relatively strong signals, and to break the lump of sawdust it was necessary to constantly hit the device. Brown recalled his old experiments with sulfide crystals, and in 1899 he recreated his old experimental setup with a new purpose - to serve as a detector of wireless signals. He used the rectification effect to convert the tiny oscillating current generated by the passing radio waves into direct current, which could be powered by a small speaker that produced audible clicks for each point or dash. This device later came to be known as the feline mustache detector.“because of the appearance of the wire, which easily touched the top of the crystal. In British India (where Bangladesh is today), the scientist and inventor Jagadish Bose built a similar device, possibly even in 1894. The rest soon began to make such detectors based on silicon and carborundum (silicon carbide).
However, it is precisely galena, lead sulfide, which has been melted to produce lead since ancient times, has become the preferred material for crystalline detectors. They turned out to be simple to manufacture and cheap, and as a result became insanely popular among the early generation of ham radio. Moreover, unlike the binary coherer (with sawdust that either went astray or not), the crystal rectifier could produce a continuous signal. Therefore, he could give out audible voice and music transmissions, and not just Morse code with its dots and dashes.
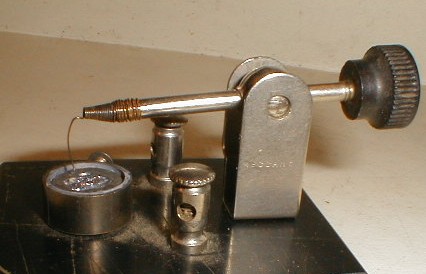
Galena-based cat whisker detector. A small piece of wire on the left is a mustache, and a piece of silver material below is a galena crystal.
However, as the annoyed hams soon discovered, it could take minutes or even hours to find a magic point on the surface of the crystal that would give a good straightening. And the signals without amplification were weak and had a metallic sound. By the 1920s, tube-based receivers with triode amplifiers had virtually eliminated crystalline detectors almost everywhere. Their attractive feature was only cheapness.
This brief appearance in the arena of radios seemed to be the limit of the practical application of the strange electrical properties of the material discovered by Brown and others.
Copper oxide
Then in the 1920s, another physicist named Lars Grondahl discovered something strange with his experimental setup. Grondal, the first of a string of smart and restless husbands in the history of the American West, was the son of a civil engineer. His father, who emigrated from Norway in 1880, worked for several decades on the railways in California, Oregon, and Washington. At first, Grondal seemed to decide to leave his father’s engineering world behind, and went to Johns Hopkins Institute for a doctorate in physics to take the academic path. But then he got involved in the railway business and took on the position of research director of Union Switch and Signal, a division of the industrial giant Westinghouse , which supplied equipment for the railway industry.
Various sources indicate conflicting reasons that motivated Grondal to study him, but be that as it may, he began experimenting with copper disks heated on one side to create an oxidized layer. Working with them, he drew attention to the asymmetry of the current - the resistance in one direction was three times greater than in the other. A disk of copper and copper oxide rectified the current, just like a sulfide crystal.

Copper oxide rectifier circuit
For the next six years, Grondal developed a commercial-use rectifier based on this phenomenon, enlisting the help of another US researcher, Paul Geiger, and then sent a patent application and announced his discovery in the American Physical Society in 1926. The device immediately became a commercial hit. Due to the absence of brittle yarns, it was much more reliable than a rectifier on electronic lamps, based on the Fleming valve principle, and was cheap in production. Unlike Brownian rectifier crystals, he worked on the first try, and thanks to the larger contact area of the metal and oxide, he worked with a wide range of currents and voltages. He could charge batteries, detect signals in various electrical systems, work as a safety shunt in powerful generators. When used as a photocell, disks could work as light meters, and were especially useful in photography. Other researchers around the same time developed selenium rectifiers that found similar uses.

A pack of rectifiers based on copper oxide. The assembly of several disks increased the reverse resistance, which allowed them to be used with high voltage.
A few years later, two physicists from Bella Laboratories, Joseph Becker and Walter Brattain , decided to study the principle of operation of a copper rectifier - they were interested to know how it works and how it can be used at Bell System.

Brattain in old age - approx. 1950
Brattain was originally from the same places as Grondal, from the Pacific Northwest, where he grew up on a farm located a few kilometers from the Canadian border. In high school, he became interested in physics, he showed abilities in this field, and eventually received his doctorate at the University of Minnesota in the late 1920s, and got a job at the Bell Laboratory in 1929. Among other things, he studied the latest theoretical physics at the university , gaining popularity in Europe, and known as quantum mechanics (its curator was John Hazbrook Van Fleck , who also instructed John Atanasov).
Quantum revolution
The new theoretical platform has been developing slowly over the past three decades, and in due time it will be able to explain all the strange phenomena that have been observed for many years in materials such as galena, selenium and copper oxide. A whole cohort of predominantly young physicists, mainly from Germany and neighboring countries, caused a quantum revolution in physics. Everywhere, look, they did not discover the smooth and continuous world that they were taught, but strange discrete lumps.
It all started in the 1890s. Max Planck, the most famous professor at the University of Berlin, decided to work with the well-known unresolved problem: how " an absolutely black body"(an ideal substance that absorbs all energy and does not reflect it) emits radiation in the electromagnetic spectrum? Various models were tried, none of which coincided with the experimental results - they could not cope either at one or the other end of the spectrum. Planck discovered that if we assume that the energy is emitted by the body in small "packets" of discrete magnitude, then we can write a simple law of the relationship between frequency and energy, which ideally matches the empirical results.
Soon after, Einstein discovered that the same thing happens with the absorption of light (the first hint of photons), and J.J. Thomson showed that electricity is also transported not by a continuous liquid or wave, but by discrete particles - electrons. Niels Bohr then created a model that explained how excited atoms emit radiation by assigning separate orbits in an atom to electrons, each of which has its own energy. However, this name is misleading because they behave in no way similar to the orbits of the planets - in the Bohr model, electrons instantly transferred from one orbit, or energy level, to another, without passing through an intermediate state. And finally, in the 1920s, Erwin Schrödinger, Werner Heisenberg, Max Bourne and others created a generalized mathematical platform known as quantum mechanics,
By this time, physicists were already convinced that materials such as selenium and galena, demonstrating photovoltaic and rectifying properties, belong to a separate class of materials, which they called semiconductors. Classification took so long for several reasons. First, the categories of “conductors” and “insulators” themselves were quite extensive. T.N. "Conductors" were extremely different in conductivity, the same (to a lesser extent) was characteristic of insulators, and it was not obvious how any particular conductor can be attributed to any of these classes. Moreover, until the middle of the 20th century it was impossible to obtain or create very pure substances, and any oddities in the conductivity of natural materials could always be attributed to pollution.
Physicists now have both mathematical tools of quantum mechanics and a new class of materials to which they could be applied. The British theoretician Alan Wilson was the first to bring it all together and built a general model of semiconductors and the principle of their work in 1931.
At first, Wilson argued that conductive materials differ from dielectrics in the state of energy zones. Quantum mechanics claims that electrons can exist on a limited number of energy levels inherent in the shells or orbitals of individual atoms. If you squeeze these atoms together in the structure of a material, then it would be more correct to imagine continuous energy zones passing through it. There are free places in conductors in high energy zones, and the electric field can freely move electrons there. In the insulators, the zones are filled, and to a higher, conductive zone, on which electricity is easier to go, to climb quite far.
This led him to conclude that impurities — foreign atoms in the structure of the material — should contribute to its semiconductor properties. They can either supply extra electrons that easily enter the conduction band, or holes — the absence of electrons compared to the rest of the material — which creates empty energy spots where free electrons can move. The first option was later called n-type semiconductors (or electronic) - for an excess negative charge, and the second - p-type, or hole - for an excess positive charge.
Finally, Wilson suggested that rectification of current by semiconductors can be explained in terms of the quantum tunneling effect., a sudden jump of electrons through a thin electric barrier in the material. The theory looked plausible, but predicted that in the rectifier, the current should flow from oxide to copper, although in reality it was the other way around.
So, despite all Wilson's breakthroughs, semiconductors remained difficult to explain. As it gradually became clear, microscopic changes in the crystal structure and concentration of impurities disproportionately affected their macroscopic electrical behavior. Ignoring the lack of understanding - since no one could explain the experimental observations made by Brown 60 years before - Brattain and Becker developed an efficient copper oxide rectifier manufacturing process for their employer. The Bell System quickly began to replace electronic lamp rectifiers throughout the system with a new device, which their engineers called a varistor , because its resistance varied depending on the direction.
gold medal
Mervyn Kelly, a physicist and former head of the Bell Laboratory’s Department of Electronic Lamps, was very interested in this achievement. For a couple of decades, electronic lamps have served Bell an invaluable service, and could perform functions inaccessible to the previous generation of mechanical and electromechanical components. But they were very hot, regularly overheated, consumed a lot of energy and were difficult to maintain. Kelly was about to rebuild the Bell system anew based on more reliable and enduring solid-state electronic components, such as a varistor, which did not require any sealed enclosures filled with gas or empty or hot filaments. In 1936, he became head of the research department at Bell Laboratories, and began to redirect the organization to a new path.
With a solid-state rectifier, the next obvious step was to create a solid-state amplifier. Naturally, like a tube amplifier, such a device could work as a digital switch. This was of particular interest to Bella, since there were still a large number of electromechanical digital switches in telephone exchanges. The company was looking for a more reliable, compact, energy-efficient, and cooler replacement of an electronic lamp in telephone systems, radios, radars, and other analog equipment, where they were used to amplify weak signals to a level accessible to the human ear.
In 1936, Bell Labs finally lifted a ban on hiring personnel introduced during the Great Depression. Kelly immediately began hiring quantum mechanics experts to help launch his solid-state device research program, including William Shockley , another West Coast native from Palo Alto, California. The theme of his recently completed MIT dissertation was the best suited to Kelly's needs: "Electronic zones in sodium chloride."
Brattain and Becker at this time continued their studies of a copper oxide rectifier, seeking to obtain an improved solid-state amplifier. The most obvious way to make it was to go by analogy with an electronic lamp. Just as Lee de Forest took a tube amp and placed an electric gridbetween the cathode and the anode, both Brattein and Becker presented how a grid can be inserted into the place where copper and copper oxide come into contact, where, as expected, rectification takes place. However, due to the small thickness of the layer, they considered it impossible to do this, and did not succeed.
Meanwhile, other developments showed that Bell Labs were not the only company interested in solid state electronics. In 1938, Rudolf Hills and Robert Paul published the results of experiments conducted at the University of Gottingen on a working solid-state amplifier created by introducing a grid into a potassium bromide crystal. This laboratory device did not represent practical value - mainly, since it worked at a frequency of not more than 1 Hz. And yet this achievement could not but rejoice all those interested in solid-state electronics. In the same year, Kelly identified Shockley as a new independent group of research on solid-state devices and gave him with colleagues - Foster Knicks and Dean Woolridge - carte blanche to study their capabilities.
At least two other inventors managed to create solid-state amplifiers before the Second World War. In 1922, the Soviet physicist and inventor Oleg Vladimirovich Losev published the results of successful experiments with zincite semiconductors, but his work went unnoticed by the Western community; in 1926, the American inventor Julius Lilenfield filed a patent for a solid-state amplifier, but there is no evidence of the operability of his invention.
Shockley’s first major inspiration in his new position occurred while reading the 1938 work of British physicist Neville Mot, Theory of Crystal Rectifiers, which finally explained the principle of operation of the Grondal rectifier on copper oxide. Mott used the mathematics of quantum mechanics to describe the formation of an electric field at the junction of a conductive metal and a semiconductor oxide, and how electrons “jump” over this electric barrier, instead of tunneling, as Wilson suggested. The current flows more easily from the metal to the semiconductor than vice versa, since the metal has much more free electrons.
This led Shockley to exactly the same idea that Brattain and Becker had considered and rejected many years before - to make a solid-state amplifier by inserting a grid of copper oxide in the gap between copper and copper oxide. He hoped that the current flowing through the grid would increase the barrier restricting the current from copper to oxide, creating an inverted, amplified version of the signal on the grid. His first gross attempt failed completely, so he turned to a man who had more refined laboratory skills and was well acquainted with rectifiers - Walter Brattain. And, although he had no doubts about the outcome, Brattain agreed to satisfy Shockley's curiosity, and created a more complex version of the “grid” amplifier. She also refused to work.
Then the war intervened, leaving Kelly's new research program in disarray. Kelly led the Bell Labs Radar Working Group, supported by MIT's main US radar research center. Brattain did not work long with him, and then went on to research the magnetic detection of submarines commissioned by the Navy. Woolridge worked on fire control systems, Knicks on gas diffusion for the Manhattan project, and Shockley went into operational research, and first engaged in the fight against submarines in the Atlantic, and then strategic bombing in the Pacific.
But, despite this intervention, the war did not stop the development of solid state electronics. On the contrary, it organized a massive infusion of resources into this area, and led to a concentration of research on two materials: germanium and silicon.
What else to read
Ernest Bruan and Stuart MacDonald, Revolution in Miniature (1978)
Friedrich Kurylo and Charles Susskind, Ferdinand Braun (1981)
GL Pearson and WH Brattain, “History of Semiconductor Research,” Proceedings of the IRE (December 1955).
Michael Riordan and Lillian Hoddeson, Crystal Fire (1997)