The Role of the IGF-1 System in Longevity Modulation: Contradictions and a New Understanding from the Perspective of Centenarians
- Transfer
Human aging is currently defined as a physiological decrease in biological functions in the body with constant adaptation to internal and external damage. The endocrine system plays an important role in the organization of cellular interactions, metabolism, growth and aging. Several studies, from worms to mishas, have shown that suppressing growth hormone / insulin-like growth factor-1 / insulin (GH / IGF-1 / insulin) pathway activity may be useful for prolonging a person's life span, while the results in humans are contradictory. In this review, we discuss the potential role of the IGF-1 system in modulating longevity, hypothesizing that endocrine and metabolic adaptation observed in centenarians and mammals with calorie restriction
Вступление.
Старение определяется как физиологическое снижение биологических функций в организме с прогрессирующим снижением или потерей адаптации к внутренним и внешним повреждениям. У людей фенотип старения чрезвычайно неоднороден и может быть описан как сложная мозаика, возникающая в результате взаимодействия нескольких случайных и экологических событий, генетических и эпигенетических изменений, накопленных в течение всей жизни. Несмотря на свою огромную сложность, молекулярная основа старения ограничена немногими высоко эволюционно консервативными биологическими механизмами, ответственными за поддержание и восстановление организма ( 1 ).
Over the past 3 decades, one of the most discussed topics in gerontology has been the role of the growth hormone (GH) / insulin-like growth factor-1 (IGF-1) / insulin system in the regulation of longevity. Accumulated data indicate that this pathway plays an important role in the pathogenesis of a number of age-related diseases, including cancer, dementia, cardiovascular and metabolic diseases (2 - 4).
In animal models, inhibition of the GH / IGF-1 / insulin system has been shown to significantly increase life span. However, in humans the data are contradictory (5, 6).
This review describes the latest advances in the study of the IGF-1 system and modulation of longevity, hypothesizes that endocrine and metabolic adaptation observed in centenarians and mammals during calorie restriction may be a physiological strategy to increase life expectancy due to a slower cell growth / metabolism, better control in signal transmission and physiological reserve capacities, and reduced accumulation of aging cells.

Fig. 1 Pleiotropic effect of IGF-1 on the body. On one side of the scale, which outweighs: tissue homeostasis, cardioprotective and neuroprotective effects, insulin-like effects, participation in the formation of the skeleton and muscle regeneration. On the second side of the balance: the risk of carcinogenesis.
The IGF-1 system has several pleiotropic effects on biological aging (see figure 1). IGF-1 plays an important role in fetal development, its growth in childhood and adolescence, and adult tissue homeostasis. In addition, IGF-1, apparently, has an atheroprotective effect, a neural protective and insulin-like effect (in high concentrations), and regulates bone metabolism and muscle regeneration. Nevertheless, IGF-1 is a major risk factor in the development of several types of tumors due to its strong proliferative activity, mainly due to cell cycle modulation, apoptosis and cell survival (7 - 9). Most of these effects are mediated by interaction with the insulin receptor substrate (IRS) -1 and-2 and the modulation of the PI3K / AKT / mammalian rapamycin target pathway (mTOR) (see Fig. 2) Fig . 2
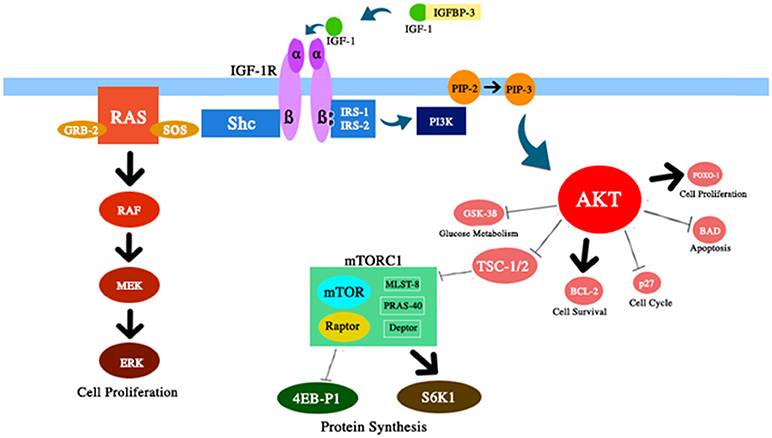
Schematic representation of several components of the IGF-1 / PI3K / AKT / mTOR path discussed in this review. IGF-1 enhances AKT activity with corresponding effects on cell survival and proliferation, glucose metabolism, and protein synthesis.
Several preclinical studies have reported that mutations in genes that control the GH / IGF-1 / insulin signaling pathway can significantly increase lifespan in both invertebrate and vertebrate animal models (5, 6).
Invertebrate Models.
In invertebrates, the insulin / IGF-like cascade is regulated by several peptides capable of interacting with one common insulin / IGF-1-like receptor.
У нематоды Caenorhabditis elegans инсулин / IGF-подобный путь состоит из нескольких белков, кодируемых генами daf-2, age-1 (кодирующий каталитическую субъединицу PI3K), akt-1, akt-2, pdk-1, sgk-1 (серин-треонинкиназы), daf-16, skn-1 и daf-18 (PTEN, фосфатаза, участвующая в ингибировании сигнального пути AKT). Было показано, что сниженная активность генов daf-2, age-1, akt-1, akt-2, pdk-1, sgk-1 подавляет этот путь, и животные с этими мутациями, как сообщалось, стареют медленнее и имеют увеличенный срок жизни до 300%. Напротив, стимуляция инсулин / IGF-подобного пути уменьшает продолжительность жизни нематод (10, 11 ).
In the fruit fly Drosophila melanogaster, insulin / IGF-like signaling consists of dINR (insulin receptor-like protein / IGF-1), CHICO insulin receptor substrate, PI3K Dp110 / p60, and PK3 PI3K target. It was reported that flies with a mutation in these genes significantly increased life expectancy (12, 13).
Surprisingly, the same molecular mechanisms in different tissues do not affect aging the same way. Several studies of nematodes and fruit flies have shown that a decrease in insulin / IGF-like signaling in nerve and adipose tissues plays a major role in regulating longevity (14, 15). Although it has been shown in invertebrate models that this cascade is important for modulating lifespan, the effects of insulin / IGF-like signaling on lifespan are much more complex in vertebrates because they have functionally specific insulin and IGF molecules, IGF binding proteins (IGFBPs ), IGFBP, GH proteases, multiple receptors, and several mechanisms of intracellular signal transmission with different tissue-specific expression (16).
Vertebrate Models
Several mutant GH / IGF-1 mice were designed with different targets. The most current models are described below.
Mice Snell and Ames.
The Snell and Ames mice are two mouse strains with mutations in the PIT-1 and PROP-1 genes, respectively (17, 18). Since both proteins PIT-1 and PROP-1 are necessary for differentiation of pituitary cells that produce GH, prolactin, and thyroid stimulating hormone, both types of homozygous mutant mice lack all three hormones (18). These models showed a significant increase in life expectancy (42–70% more than in wild-type mice), increased insulin sensitivity and a lower tumor frequency (19, 20). When Ames mice were restricted in calories, their lifespan increased even more (21). Although these animals lack three hormones, it has been shown that increasing longevity mainly depends on GH deficiency (22).
Lit / lit mouse
Lit / lit mice have GH deficiency and a mutation in the gene that encodes the GH releasing hormone receptor (GHRHR). These animals were dwarfs, had increased adipose tissue, lower tumor rates, and life expectancy increased by 23–25% (19).
GH-Releasing Hormone-Knockout (GHRH-KO) mouse.
Knockout mice GH-releasing hormone (GHRH-KO) live 43% (in females) and 51% (in males) longer than wild-type animals and have many phenotypic characteristics of Ames mice, such as increased sensitivity to insulin, a decrease in plasma triglycerides and cholesterol levels, an increase in adipose tissue, elevated plasma leptin and adiponectin levels (23).
GH-Receptor-Knockout Mice (GHR-KO)
GH receptor knockout mice (GHR-KO) have elevated serum GH levels and very low IGF-1 levels. It was also reported that this strain of mice lived 38–55% longer than the wild type (24) and showed a reduction in oxidative stress, as well as a lower and delayed onset of fatal tumors (25). Similar results were observed in df / KO mice, crossed two strands of misha, GHR-KO and Ames dwarfs, which lacked GH and GH receptor and continued to increase life expectancy (26). Unlike wild Ames siblings and dwarf mice, calorie restriction did not increase the lifespan of GHR-KO mice, suggesting that the GH / IGF-1 axis and calorie restriction may have similar or partially overlapping mechanisms to extend lifespan (27) .
GH receptor antagonism (GHA)
Not all animal models with suppression of the GH / IGF-1 system show an increase in life expectancy. The GHA strain of mice is one such example. GHA, generated by replacing one amino acid, is able to bind the GH receptor with the same affinity as GH, but does not cause intracellular signaling. Life expectancy of GHA mice did not significantly increase (28).
IGF-1R +/– Mice
While most mice with an inactive IGF-1 receptor (IGF-1R - / -) die at birth, animals heterozygous for the mutant allele of the IGF-1 receptor (IGF-1R ±) showed very low levels of serum IGF-1 , about 10% smaller and 33% longer life expectancy in females and 16% in males. However, in this study, wild-type controls survived to 19 months, jeopardizing the interpretation of the results (29). More recent studies evaluating life expectancy in another IGF-1R ± line showed a slight increase in life expectancy by 5–10%, but only in females (30, 31). In addition, the main background strain seems to affect the degree of life extension in several mouse models (32).
A Brain-Specific IGF1-R +/−
A Brain-Specific IGF1-R +/− mutant mice lived 9% longer than the wild type, which emphasizes the corresponding role of the nervous system in modulating longevity (33).
Liver-specific IGF-1-disrupted mice (LI-IGF-1 - / -).
Mice with impaired IGF-1 production (LI-IGF-1 - / -) have very low serum levels of IGF-1 and high serum GH levels due to inactivation of the IGF-1 gene. LI-IGF-1 - / - mice had markedly reduced obesity and, as a result, the weight was 25% lower than in wild-type mice. Only female LI-IGF-1 - / - mice showed an increase in life expectancy of 16% compared to control mice (34).
Pappa - / - mice.
Pappa - / - mice have a knockout of the gene for Pregnancy-associated plasma protein A, PAPP-A, PAPPA, a specific protease for IGF binding proteins. The average lifespan of this mouse strain was 38% longer compared to wild-type controls. Pappa - / - mice were dwarfs, but their serum glucose, insulin, IGF-1, and GH levels did not differ from those of the wild-type controls, suggesting that PAPPA acts primarily at the autocrine or paracrine level. In addition to increasing life expectancy, Pappa - / - mice showed a lower incidence of tumors, as well as age-related degenerative lesions (35, 36).
IRS Disrupted (IRS1 - / -) mouse.
IRS-1 and -2 are important mediators for insulin as well as for IGF-1 signaling. IRS1 - / - mice were insulin-resistant, with a defect in the transmission of insulin signals mainly in muscle tissue, about 30% shorter than wild-type, and only females had an 18% longer life expectancy compared to wild-type animals (37 ) )
IRS2 - / - mice were also insulin resistant, but unlike IRS1 - / - mice, they showed insulin signaling defects in more tissues, including liver, adipose tissue, and skeletal muscle. These mice developed diabetes, and their lifespan was much shorter than that of wild-type mice and IRS2 ± mice. IRS2 +/− mice had improved insulin sensitivity and increased life expectancy (+ 18%) compared with wild-type mice. In addition, brain-specific mice IRS2 ± and IRS2 - / - were reported to be insulin resistant and lived 18 and 14% longer than wild-type controls, respectively (38).
KLOTHO Modified Mice.
The KLOTHO protein inhibits insulin and IGF-1 signaling, possibly by disrupting the receptor / ligand interaction. It was reported that mice with KLOTHO overexpression were of normal size, and insulin resistance developed in males, and life expectancy in both males and females was significantly increased (+18 and + 30%, respectively) (39, 40).
P66shc Disrupted Mouse (P66shc - / -).
P66shc is a protein that mediates IGF-1 signaling after the receptor by activating the MAPK pathway. P66shc - / - mice had a normal phenotype, but lived 28% longer than wild-type controls (41). However, these data were not confirmed in a recent study (42).
The role of GH / IGF-1 / insulin signaling in aging and longevity has been deeply studied in all of these animal models. While invertebrates, the effect of suppression of the IGF-1 / insulin pathway on life expectancy was obvious and significant, in mouse models this effect was weakened and not reproducible in some cases, such as in the IGF-1R ± and P66shc - / - lines mice. However, most of these models showed some common features among long-living mice, such as reduced levels of circulating IGF-1 and insulin and increased insulin sensitivity, which are likely to reduce cancer incidence, improve stress resistance and prolong life. . Genetic changes that can disrupt the IGF-1 system, can keep animals healthy for longer periods and can delay or alleviate some age-related diseases. In this process, the nervous and fatty tissues play an important role.
In addition, additional data is needed to determine the best point in time during the life span to intervene in the suppression of the IGF-1 system to obtain a positive effect on life span. In igf f / f C57B l / 6 mice, a deficiency of circulating IGF-1, starting from 5 months of age or earlier, increased life expectancy by 15% only in females with a decrease in the number of organs with pathology at the end of life compared to the control group . In addition, late IGF-1 deficiency (15 months) reduced the risk of cancer, but did not have a positive effect on life expectancy (43). These data underline the importance of IGF-1 deficiency at an early age to increase life expectancy. On the other hand, Mao et al. (44) recently reported
Centennial centenarians are considered the best human model for studying the biological determinants of longevity, which have reached the most extreme values of human life expectancy (45).
Several studies compared circulating insulin and IGF-1 levels in long-livers with those in younger controls (46).
Metabolic age-related remodeling is a physiological process that occurs in the entire population. Aging is often associated with a decrease in glucose tolerance, which is associated with increased insulin resistance (47), but long-lived people have an exception. It was found (48) that insulin resistance increases with age and decreases in people older than 90 years living in southern Italy. Indeed, long-lived subjects showed higher insulin sensitivity and better beta-cell function than younger subjects. This difference also did not depend on the main anthropometric and metabolic factors. In 100-year-old patients, the plasma glucose concentration for 2 hours was lower than in the elderly (average age 78 years). In long-livers, insulin-mediated glucose intake was higher than in elderly controls during euglycemic glucose uptake, which maintained the preserved glucose tolerance and insulin action in this long-living group (49, 50). Similar results confirming the best sensitivity to insulin were observed in other populations of long-livers (51, 52).
In addition, long-livers showed the preserved effect of insulin not only on glucose metabolism, but also on adipose tissue. In fact, insulin infusion is usually associated with inhibition of lipolysis and, thus, with a significant decrease in the concentration of free fatty acids and triglycerides in plasma. In long-livers, the inhibitory activity of insulin during lipolysis was higher than in the control participants (mean age 78 years) (50). It should be noted that in long-livers, in comparison with adult controls, lower sympathetic tone is also observed, which may be associated with a better action of insulin and, therefore, with a low level of fasting insulin in plasma (53, 54).
The data on the IGF-1 system regarding life expectancy are still contradictory in long-lived subjects (46). Paolisso et al. (55) described an increased plasma IGF-1 / IGFBP-3 ratio in healthy long-livers compared with older people. They suggested that this increased ratio indicates a higher bioavailability of IGF-1, which contributes to the improvement of insulin action in long-livers. Bonafè et al. (56) reported that subjects with at least the A allele of the IGF-1 receptor gene (G / A, codon 1013) had low plasma levels of free IGF-1 and were more prevalent among long-lived individuals. Arai et al. (57) described relatively low levels of serum IGF-1 in the Japanese long-livers population. In this population, the lowest rates of both IGF-1 and IGFBP-3 were associated with increased mortality (58).
These conflicting results probably reflect the complexity of the IGF system and ethnic differences in the registered population. In addition, centenarians were often compared with a control group of younger subjects. Thus, in most of these studies, it was impossible to conclude whether the differences between IGF-1 in both groups were associated with different life expectancy or reflected a physiologically age-dependent decrease in IGF-1. Indeed, there are several limitations to the study of long-livers: (1) low prevalence (1 centenary per 5-10,000 inhabitants), (2) weakness due to extreme age (almost 95% of long-livers have at least 1 asthenia criterion), (3 ) the absence of a control group of the same age (45, 59). Due to these limitations, this human model is not suitable for studying age-related variables,
The descendants of centenarians are another interesting model for determining the relevant factors associated with human longevity and healthy aging. A consistent set of observations in different countries suggests that the descendants of long-livers are healthier than representatives of the same demographic groups (51, 60, 61) and biologically (epigenetically) younger than their chronological age (62). In general, these studies show that relatives of centenarians are more likely to live longer and have good health (60, 63). In addition, the study of the offspring of long-livers has the advantage of having an appropriate demographically selected control group consisting of a progeny of comparable age, in which both parents were born in the same cohort of centenarians, but died before the threshold age. after which people are classified as “centenarians.” This strategy is critical to preventing cohort effects. Thus, the model of offspring of long-livers can overcome some of the limitations that are found in the study of long-livers (rarity, weakness, and lack of proper control) (60).
In several studies, the IGF-1 / insulin system was characterized in descendants of long-livers and in the corresponding control group.
We evaluated the circulating bioactivity of IGF-1, measured using an innovative kinase receptor activation assay (KIRA) performed on long-livers, long-livers descendants, and comparable control offspring. The offspring of long-livers and long-livers had a relatively lower circulating biological activity of IGF-1 compared to the control group. Interestingly, the biological activity of IGF-1 in descendants of long-livers was inversely related to insulin sensitivity (51).
Suh et al. ( 64 ) оценивали уровни IGF-1 в сыворотке у потомков еврейских долгожителей ашкенази и в сопостаимых по возрасту контролях. У детей долгожителей женского пола уровень IGF-1 в сыворотке был на 35% выше, чем у контрольних участников. Эта разница может представлять компенсаторный ответ на снижение передачи сигналов рецептора IGF-1. Кроме того, у еврейских долгожителей ашкенази было описано чрезмерное присутствие гетерозиготных мутаций в гене рецептора IGF-1 вместе с относительно высокими уровнями IGF-1 в сыворотке и ослабленной активностью рецептора IGF-1 по сравнению с контрольной группой без семейного долголетия.
To study longevity, other authors examined ninety-year-old brothers and sisters and their descendants. In the Leyden study of longevity, 421 families were selected, consisting of at least two reporters, brothers and sisters, their descendants and partners of descendants as a control. In these populations, serum glucose, insulin, and triglycerides were the best biomarker for healthy aging (low glucose and insulin levels were considered healthy) (65). Nine-year-old centenarians in the lowest IGF-1 / IGFBP-3 ratio in the bloodstream were associated with better survival (66). Descendants of nine-year-olds showed better insulin sensitivity compared to their partners, while similar levels of fasting serum IGF-1 and IGFBP-3 were observed in both fasting groups (67). Interesting,
Another approach taken to study longevity in humans is to select family components for exceptional longevity and healthy aging based on strict criteria, such as the Long Life Family Study, a measure of family life span. These families, selected for exceptional life expectancy, were compared with a control group without a family history of longevity (69). In this population, circulating levels of IGF-1 proved to be a reliable age-related biomarker (70).
In support of the potential role of the GH / IGF-1 / insulin system in human longevity, there are many genetic studies. Indeed, it has been found that several genetic loci are associated with circulating levels of IGF-1 and IGFBP-3 and are potentially capable of affecting aging (71). A genome-wide analysis of associations conducted in ninety-year-olds and patient populations aged <60 years showed a clear link between the genetic variation of genes involved in the insulin / IGF-1 pathway and human lifespan (72). In a prospective study of older people, women with a genetic profile, indicating a decrease in insulin / IGF-1 signaling activity, showed longer life spans (73). In four independent cohorts of long-lived individuals, a linear increase in the prevalence of exogenous 3 (G3R) GH receptor homozygosity with age has recently been described. The presence of the d3 / d3 genotype increased life expectancy by about 10 years (74).
One of the most convincing observations in the biology of aging is the ability to limit calories to prevent or delay some age-related diseases and increase the life expectancy of mammals (75 - 78). The biological mechanisms of this phenomenon are not entirely clear, but the suggestion was made about the potential involvement of corresponding changes in energy metabolism, the endocrine system and oxidative damage.
Calorie restriction causes numerous hormonal changes. In rodents, caloric restriction without starvation suppressed circulating levels of IGF-1 and insulin in proportion to the level of restriction, increased insulin sensitivity and resistance to stress and toxicity, and reduced the risk of cancer (79, 80). Interestingly, most of these characteristics observed in wild-type mice during caloric restriction resemble those reported in mice that are long-living due to genetic impairment of GH / IGF-1 / insulin signaling, as described previously.
Randomized clinical trials in humans have shown that calorie restriction does not reduce serum IGF-1 unless protein intake is reduced (81, 82). However, a recent meta-analysis evaluating the effect of nutritional restriction on the recognized biomarkers of healthy aging has shown a decrease in human IGF-1 levels in blood (83).
Other hormonal changes, such as a decrease in insulin levels, thyroid hormones and leptin levels, as well as an increase in adiponectin and insulin sensitivity, were observed during diet restriction (85, 86).
This hormonal adaptation can play an important role in prolonging life through several mechanisms:
1) Decreased metabolic rate, cell proliferation and oxidative stress. In fact, IGF-1 is a potent growth factor, and thyroid hormone is a potent stimulator of metabolic rate and metabolic rate. In addition, transcription patterns suggest that chronic moderate calorie restriction in adults slows down the aging process, switching cell metabolism from growth to maintenance and recovery (84).
2) Reducing the accumulation of aging cells. Cell aging has been shown to be a key mediator of aging (87). Over time, protein homeostasis decreases and damage accumulates. Interestingly, some age-related diseases can be delayed by weakening the accumulation of aging cells (88, 89). Typically, the mTOR pathway is activated by several signals, including nutrients, IGF-1, and insulin (Fig. 2). The deregulation of this pathway, which was reported after calorie restriction, increased the lifespan of some organisms. This effect, apparently, is secondary to an increase in autophagy, a cytoprotective digestion process. In fact, autophagy is a cell processing process that can remove old or damaged cellular components, preventing the accumulation of aging cells (90,
3) Counteracting inflammation. Dietary intervention in both animals and humans can slow the aging process, weakening the inflammatory status of a weak degree (83, 92). The mechanisms underlying the anti-inflammatory activity of nutritional restriction are not clearly defined. It is believed that this effect is due to a decrease in fat mass and proinflammatory adipokines, as well as an improvement in the integrity of the intestinal barrier observed during dietary intervention (93, 94).
Интересно отметить, что эндокринный биохимический профиль, наблюдаемый у субъектов во время ограничения калорийности, сопоставим с таковым у долгожителей, что подтверждает потенциальную роль эндокринной системы в модуляции продолжительности жизни. В дополнение к увеличению чувствительности к инсулину и снижению уровней IGF-1 в плазме / сыворотке, в нескольких исследованиях было показано повышение уровня циркулирующего адипонектина и снижение уровней циркулирующих лептина и тиреоидных гормонов у долгоживущих людей по сравнению с более молодыми субъектами.
Adipose tissue is an endocrine organ that produces several cytokines involved in relevant processes, such as energy metabolism, lipid and glucose homeostasis, and modulation of the inflammatory response. Visceral adipose tissue plays a major role in the development of metabolic diseases (95). Aging is associated with an increase in fat mass and redistribution of adipose tissue, characterized by a loss of peripheral subcutaneous fat and an accumulation of visceral fat. In older people, changes in the secretion, synthesis and function of adipokines have been described, probably due to an imbalance in the function, proliferation, size and number of fat cells (86). Adiponectin is an insulin-sensitizing, anti-inflammatory and anti-atherogenic cytokine. Adiponectin circulates in the blood in several forms: trimer, hexamer, high molecular weight multimer (HMW) and globular adiponectin (proteolytically cleaved form). The multimer HMW is believed to be a more active form of adiponectin for protection against insulin resistance and diabetes (96). Circulating adiponectin is independently and negatively associated with aspects of the metabolic syndrome, including insulin resistance, body weight, blood pressure and serum lipids. Leptin is mainly produced in the subcutaneous and to a lesser extent in the visceral white adipose tissue. This cytokine regulates food intake, energy expenditure, and atherogenesis. Leptin promotes weight loss by reducing appetite and stimulating metabolism and has pro-inflammatory properties (97). that the multimer HMW is a more active form of adiponectin in protection against insulin resistance and diabetes (96). Circulating adiponectin is independently and negatively associated with aspects of the metabolic syndrome, including insulin resistance, body weight, blood pressure and serum lipids. Leptin is mainly produced in the subcutaneous and to a lesser extent in the visceral white adipose tissue. This cytokine regulates food intake, energy expenditure, and atherogenesis. Leptin promotes weight loss by reducing appetite and stimulating metabolism and has pro-inflammatory properties (97). that the multimer HMW is a more active form of adiponectin in protection against insulin resistance and diabetes (96). Circulating adiponectin is independently and negatively associated with aspects of the metabolic syndrome, including insulin resistance, body weight, blood pressure and serum lipids. Leptin is mainly produced in the subcutaneous and to a lesser extent in the visceral white adipose tissue. This cytokine regulates food intake, energy expenditure, and atherogenesis. Leptin promotes weight loss by reducing appetite and stimulating metabolism and has pro-inflammatory properties (97). blood pressure and serum lipids. Leptin is mainly produced in the subcutaneous and to a lesser extent in the visceral white adipose tissue. This cytokine regulates food intake, energy expenditure, and atherogenesis. Leptin promotes weight loss by reducing appetite and stimulating metabolism and has pro-inflammatory properties (97). blood pressure and serum lipids. Leptin is mainly produced in the subcutaneous and to a lesser extent in the visceral white adipose tissue. This cytokine regulates food intake, energy expenditure, and atherogenesis. Leptin promotes weight loss by reducing appetite and stimulating metabolism and has pro-inflammatory properties (97).
Several studies have reported that long-livers have higher plasma adiponectin levels and lower leptin concentrations than younger controls (53, 98 - 102). All forms of adiponectin were significantly increased in long-livers, but the multimer HMW was significantly higher (99). In long-livers, high adiponectin concentrations turned out to be independent of BMI, renal or cardiovascular function and were associated with a favorable metabolic phenotype (higher levels of HDL-C, lower glycated hemoglobin, insulin, HOMA-IR and triglycerides) (98, 99). Elevated adiponectin levels have also been found in offspring of long-lived subjects (over 95 years old) (103).
A decrease in the level of thyroid hormones is apparently characteristic of long-livers. Mariotti et al. (104) reported that healthy centenarians had lower serum TSH and FT3 levels and higher serum rT3 levels compared to those observed in other control groups. In another Italian population of long-livers, the total T4 values were below the normal range in 60% of the examined (105). Baranovskaya et al. reported that serum T3 levels in centenarians were lower than those in early elderly and young women (52). We recently characterized the thyroid function profile in an Italian cohort of 672 patients (aged 52–113 years). An age-related decrease in FT3 level and FT3 / FT4 ratios were noted, while FT4 and TSH increase with age (106).
Корсонелло и соавт. ( 108 ) обнаружили у родственников долгожителей (потомство или племянницы / племянники) более низкие сопутствующие заболевания, уровни FT3, FT4 и TSH, чем у сопоставимых по возрасту контролей, которые не были родственниками долгожителей. В другой итальянской популяции более низкий уровень FT4 в плазме наблюдался у потомков долгожителей по сравнению с контрольной группой того же возраста ( 60 ).
In general, long-livers are thin (109) and adhere to healthy eating habits (110). Similar to subjects during caloric restriction, long-livers observed slower cell growth / metabolism, better control of signal transmission and increased autophagy. Through analysis of DNA methylation throughout the genome in centenarians and their descendants, we have identified epigenetically modulated genes and pathways that are potentially involved in aging and longevity. Our results indicate that these populations were characterized by better conservation of DNA methylation status, slower cell growth / metabolism and better control of signal transmission through epigenetic mechanisms (111). In long-livers, bioenergetic function is preserved due to mitochondrial hypertrophy, which can compensate for functional defects (112). In addition, healthy long-livers have high levels of autophagy, as evidenced by higher serum beclin-1 levels in comparison with both young patients with myocardial infarction and healthy controls (113). An increase in autophagic activity was also observed in subjects belonging to families with exceptional longevity (114).
The corresponding phenomenon arises in relation to the inflammatory status, which weakens in subjects after calorie restriction (115, 116) and is high in long-livers (117 - 119). With aging, there is a state of mild and chronic inflammatory pathology (age-related inflammation) and an increased prevalence of a number of diseases, such as cardiovascular diseases, atherosclerosis, tumors, cognitive impairment, osteoarthritis and diabetes (120, 121). Therefore, the weakening of chronic inflammatory status after calorie restriction is a beneficial effect. Centennial centenarians show signs of inflammation, but at the same time, it seems, spared its harmful effects. This obvious paradox can be explained by the fact
These data suggest the general mechanisms for increasing longevity and delaying age-related diseases found in long-livers and mammals during a calorie-restricted diet.
Opinion of the authors.
Preclinical models have provided a deeper insight into the aging process using consistent data that takes into account the role of the GH / IGF-1 / insulin system in modulating life expectancy. Although it is well known that increased insulin sensitivity and low insulin levels are associated with improved survival, there is some evidence showing that a weakening of the GH / IGF-1 axis can have a beneficial effect on increasing life expectancy in humans. However, it is still unknown what are the optimal levels of IGF-1 throughout life to live longer and be healthier. In addition, IGF-1 receptor sensitivity and activation of the post-receptor pathway have not been evaluated in most studies involving long-lived subjects. Consequently, it is not possible to determine the actual state of IGF-1 receptor signaling activation by a simple dose of circulating levels of IGF-1. This makes it difficult to identify pharmacological or environmental strategies that target this system to increase longevity and promote healthy aging. A comprehensive understanding of these aspects remains the main problem for identifying interventions aimed at slowing down human aging and for use in rehabilitation medicine. Future studies should evaluate the functional state of IGF-1 receptor signaling, including through transcriptional profiling and analysis of functional networks regarding IGF-1 regulated genes in long-lived subjects. This makes it difficult to identify pharmacological or environmental strategies that target this system to increase longevity and promote healthy aging. A comprehensive understanding of these aspects remains the main problem for identifying interventions aimed at slowing down human aging and for use in rehabilitation medicine. Future studies should evaluate the functional state of IGF-1 receptor signaling, including through transcriptional profiling and analysis of functional networks regarding IGF-1 regulated genes in long-lived subjects. This makes it difficult to identify pharmacological or environmental strategies that target this system to increase longevity and promote healthy aging. A comprehensive understanding of these aspects remains the main problem for identifying interventions aimed at slowing down human aging and for use in rehabilitation medicine. Future studies should evaluate the functional state of IGF-1 receptor signaling, including through transcriptional profiling and analysis of functional networks regarding IGF-1 regulated genes in long-lived subjects. and applications in rehabilitation medicine. Future studies should evaluate the functional state of IGF-1 receptor signaling, including through transcriptional profiling and analysis of functional networks regarding IGF-1 regulated genes in long-lived subjects. and applications in rehabilitation medicine. Future studies should evaluate the functional state of IGF-1 receptor signaling, including through transcriptional profiling and analysis of functional networks regarding IGF-1 regulated genes in long-lived subjects.
Conclusions A
striking similarity has been described with respect to the endocrine profile between long-livers and subjects after a calorie-restricted diet. The endocrine and metabolic adaptation observed in both models can be a physiological strategy for increasing life expectancy due to slower cell growth / metabolism, slower loss of the natural physiological reserve, the transition of cellular metabolism from cell proliferation to regenerative activity, and reducing the accumulation of aging cells. Apparently, these mechanisms, at least partially, are mediated by modulation of the GH / IGF-1 / insulin system.
Вступление.
Старение определяется как физиологическое снижение биологических функций в организме с прогрессирующим снижением или потерей адаптации к внутренним и внешним повреждениям. У людей фенотип старения чрезвычайно неоднороден и может быть описан как сложная мозаика, возникающая в результате взаимодействия нескольких случайных и экологических событий, генетических и эпигенетических изменений, накопленных в течение всей жизни. Несмотря на свою огромную сложность, молекулярная основа старения ограничена немногими высоко эволюционно консервативными биологическими механизмами, ответственными за поддержание и восстановление организма ( 1 ).
Over the past 3 decades, one of the most discussed topics in gerontology has been the role of the growth hormone (GH) / insulin-like growth factor-1 (IGF-1) / insulin system in the regulation of longevity. Accumulated data indicate that this pathway plays an important role in the pathogenesis of a number of age-related diseases, including cancer, dementia, cardiovascular and metabolic diseases (2 - 4).
In animal models, inhibition of the GH / IGF-1 / insulin system has been shown to significantly increase life span. However, in humans the data are contradictory (5, 6).
This review describes the latest advances in the study of the IGF-1 system and modulation of longevity, hypothesizes that endocrine and metabolic adaptation observed in centenarians and mammals during calorie restriction may be a physiological strategy to increase life expectancy due to a slower cell growth / metabolism, better control in signal transmission and physiological reserve capacities, and reduced accumulation of aging cells.
IGF-1 system and durability in animal models

Fig. 1 Pleiotropic effect of IGF-1 on the body. On one side of the scale, which outweighs: tissue homeostasis, cardioprotective and neuroprotective effects, insulin-like effects, participation in the formation of the skeleton and muscle regeneration. On the second side of the balance: the risk of carcinogenesis.
The IGF-1 system has several pleiotropic effects on biological aging (see figure 1). IGF-1 plays an important role in fetal development, its growth in childhood and adolescence, and adult tissue homeostasis. In addition, IGF-1, apparently, has an atheroprotective effect, a neural protective and insulin-like effect (in high concentrations), and regulates bone metabolism and muscle regeneration. Nevertheless, IGF-1 is a major risk factor in the development of several types of tumors due to its strong proliferative activity, mainly due to cell cycle modulation, apoptosis and cell survival (7 - 9). Most of these effects are mediated by interaction with the insulin receptor substrate (IRS) -1 and-2 and the modulation of the PI3K / AKT / mammalian rapamycin target pathway (mTOR) (see Fig. 2) Fig . 2
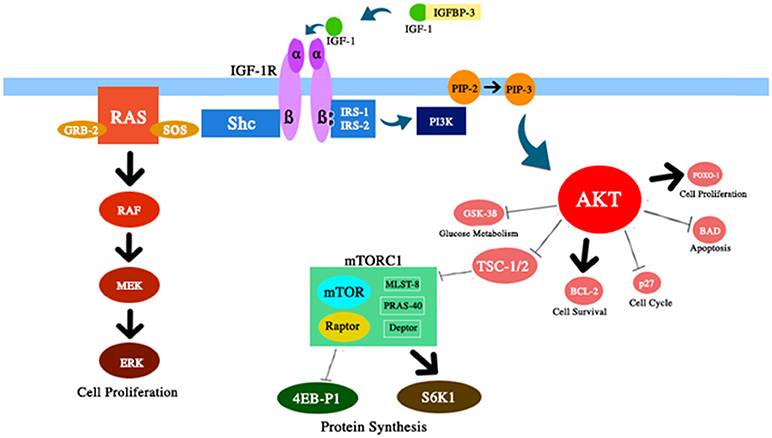
Schematic representation of several components of the IGF-1 / PI3K / AKT / mTOR path discussed in this review. IGF-1 enhances AKT activity with corresponding effects on cell survival and proliferation, glucose metabolism, and protein synthesis.
Several preclinical studies have reported that mutations in genes that control the GH / IGF-1 / insulin signaling pathway can significantly increase lifespan in both invertebrate and vertebrate animal models (5, 6).
Invertebrate Models.
In invertebrates, the insulin / IGF-like cascade is regulated by several peptides capable of interacting with one common insulin / IGF-1-like receptor.
У нематоды Caenorhabditis elegans инсулин / IGF-подобный путь состоит из нескольких белков, кодируемых генами daf-2, age-1 (кодирующий каталитическую субъединицу PI3K), akt-1, akt-2, pdk-1, sgk-1 (серин-треонинкиназы), daf-16, skn-1 и daf-18 (PTEN, фосфатаза, участвующая в ингибировании сигнального пути AKT). Было показано, что сниженная активность генов daf-2, age-1, akt-1, akt-2, pdk-1, sgk-1 подавляет этот путь, и животные с этими мутациями, как сообщалось, стареют медленнее и имеют увеличенный срок жизни до 300%. Напротив, стимуляция инсулин / IGF-подобного пути уменьшает продолжительность жизни нематод (10, 11 ).
In the fruit fly Drosophila melanogaster, insulin / IGF-like signaling consists of dINR (insulin receptor-like protein / IGF-1), CHICO insulin receptor substrate, PI3K Dp110 / p60, and PK3 PI3K target. It was reported that flies with a mutation in these genes significantly increased life expectancy (12, 13).
Surprisingly, the same molecular mechanisms in different tissues do not affect aging the same way. Several studies of nematodes and fruit flies have shown that a decrease in insulin / IGF-like signaling in nerve and adipose tissues plays a major role in regulating longevity (14, 15). Although it has been shown in invertebrate models that this cascade is important for modulating lifespan, the effects of insulin / IGF-like signaling on lifespan are much more complex in vertebrates because they have functionally specific insulin and IGF molecules, IGF binding proteins (IGFBPs ), IGFBP, GH proteases, multiple receptors, and several mechanisms of intracellular signal transmission with different tissue-specific expression (16).
Vertebrate Models
Several mutant GH / IGF-1 mice were designed with different targets. The most current models are described below.
Mice Snell and Ames.
The Snell and Ames mice are two mouse strains with mutations in the PIT-1 and PROP-1 genes, respectively (17, 18). Since both proteins PIT-1 and PROP-1 are necessary for differentiation of pituitary cells that produce GH, prolactin, and thyroid stimulating hormone, both types of homozygous mutant mice lack all three hormones (18). These models showed a significant increase in life expectancy (42–70% more than in wild-type mice), increased insulin sensitivity and a lower tumor frequency (19, 20). When Ames mice were restricted in calories, their lifespan increased even more (21). Although these animals lack three hormones, it has been shown that increasing longevity mainly depends on GH deficiency (22).
Lit / lit mouse
Lit / lit mice have GH deficiency and a mutation in the gene that encodes the GH releasing hormone receptor (GHRHR). These animals were dwarfs, had increased adipose tissue, lower tumor rates, and life expectancy increased by 23–25% (19).
GH-Releasing Hormone-Knockout (GHRH-KO) mouse.
Knockout mice GH-releasing hormone (GHRH-KO) live 43% (in females) and 51% (in males) longer than wild-type animals and have many phenotypic characteristics of Ames mice, such as increased sensitivity to insulin, a decrease in plasma triglycerides and cholesterol levels, an increase in adipose tissue, elevated plasma leptin and adiponectin levels (23).
GH-Receptor-Knockout Mice (GHR-KO)
GH receptor knockout mice (GHR-KO) have elevated serum GH levels and very low IGF-1 levels. It was also reported that this strain of mice lived 38–55% longer than the wild type (24) and showed a reduction in oxidative stress, as well as a lower and delayed onset of fatal tumors (25). Similar results were observed in df / KO mice, crossed two strands of misha, GHR-KO and Ames dwarfs, which lacked GH and GH receptor and continued to increase life expectancy (26). Unlike wild Ames siblings and dwarf mice, calorie restriction did not increase the lifespan of GHR-KO mice, suggesting that the GH / IGF-1 axis and calorie restriction may have similar or partially overlapping mechanisms to extend lifespan (27) .
GH receptor antagonism (GHA)
Not all animal models with suppression of the GH / IGF-1 system show an increase in life expectancy. The GHA strain of mice is one such example. GHA, generated by replacing one amino acid, is able to bind the GH receptor with the same affinity as GH, but does not cause intracellular signaling. Life expectancy of GHA mice did not significantly increase (28).
IGF-1R +/– Mice
While most mice with an inactive IGF-1 receptor (IGF-1R - / -) die at birth, animals heterozygous for the mutant allele of the IGF-1 receptor (IGF-1R ±) showed very low levels of serum IGF-1 , about 10% smaller and 33% longer life expectancy in females and 16% in males. However, in this study, wild-type controls survived to 19 months, jeopardizing the interpretation of the results (29). More recent studies evaluating life expectancy in another IGF-1R ± line showed a slight increase in life expectancy by 5–10%, but only in females (30, 31). In addition, the main background strain seems to affect the degree of life extension in several mouse models (32).
A Brain-Specific IGF1-R +/−
A Brain-Specific IGF1-R +/− mutant mice lived 9% longer than the wild type, which emphasizes the corresponding role of the nervous system in modulating longevity (33).
Liver-specific IGF-1-disrupted mice (LI-IGF-1 - / -).
Mice with impaired IGF-1 production (LI-IGF-1 - / -) have very low serum levels of IGF-1 and high serum GH levels due to inactivation of the IGF-1 gene. LI-IGF-1 - / - mice had markedly reduced obesity and, as a result, the weight was 25% lower than in wild-type mice. Only female LI-IGF-1 - / - mice showed an increase in life expectancy of 16% compared to control mice (34).
Pappa - / - mice.
Pappa - / - mice have a knockout of the gene for Pregnancy-associated plasma protein A, PAPP-A, PAPPA, a specific protease for IGF binding proteins. The average lifespan of this mouse strain was 38% longer compared to wild-type controls. Pappa - / - mice were dwarfs, but their serum glucose, insulin, IGF-1, and GH levels did not differ from those of the wild-type controls, suggesting that PAPPA acts primarily at the autocrine or paracrine level. In addition to increasing life expectancy, Pappa - / - mice showed a lower incidence of tumors, as well as age-related degenerative lesions (35, 36).
IRS Disrupted (IRS1 - / -) mouse.
IRS-1 and -2 are important mediators for insulin as well as for IGF-1 signaling. IRS1 - / - mice were insulin-resistant, with a defect in the transmission of insulin signals mainly in muscle tissue, about 30% shorter than wild-type, and only females had an 18% longer life expectancy compared to wild-type animals (37 ) )
IRS2 - / - mice were also insulin resistant, but unlike IRS1 - / - mice, they showed insulin signaling defects in more tissues, including liver, adipose tissue, and skeletal muscle. These mice developed diabetes, and their lifespan was much shorter than that of wild-type mice and IRS2 ± mice. IRS2 +/− mice had improved insulin sensitivity and increased life expectancy (+ 18%) compared with wild-type mice. In addition, brain-specific mice IRS2 ± and IRS2 - / - were reported to be insulin resistant and lived 18 and 14% longer than wild-type controls, respectively (38).
KLOTHO Modified Mice.
The KLOTHO protein inhibits insulin and IGF-1 signaling, possibly by disrupting the receptor / ligand interaction. It was reported that mice with KLOTHO overexpression were of normal size, and insulin resistance developed in males, and life expectancy in both males and females was significantly increased (+18 and + 30%, respectively) (39, 40).
P66shc Disrupted Mouse (P66shc - / -).
P66shc is a protein that mediates IGF-1 signaling after the receptor by activating the MAPK pathway. P66shc - / - mice had a normal phenotype, but lived 28% longer than wild-type controls (41). However, these data were not confirmed in a recent study (42).
The role of GH / IGF-1 / insulin signaling in aging and longevity has been deeply studied in all of these animal models. While invertebrates, the effect of suppression of the IGF-1 / insulin pathway on life expectancy was obvious and significant, in mouse models this effect was weakened and not reproducible in some cases, such as in the IGF-1R ± and P66shc - / - lines mice. However, most of these models showed some common features among long-living mice, such as reduced levels of circulating IGF-1 and insulin and increased insulin sensitivity, which are likely to reduce cancer incidence, improve stress resistance and prolong life. . Genetic changes that can disrupt the IGF-1 system, can keep animals healthy for longer periods and can delay or alleviate some age-related diseases. In this process, the nervous and fatty tissues play an important role.
In addition, additional data is needed to determine the best point in time during the life span to intervene in the suppression of the IGF-1 system to obtain a positive effect on life span. In igf f / f C57B l / 6 mice, a deficiency of circulating IGF-1, starting from 5 months of age or earlier, increased life expectancy by 15% only in females with a decrease in the number of organs with pathology at the end of life compared to the control group . In addition, late IGF-1 deficiency (15 months) reduced the risk of cancer, but did not have a positive effect on life expectancy (43). These data underline the importance of IGF-1 deficiency at an early age to increase life expectancy. On the other hand, Mao et al. (44) recently reported
IGF-1 system in long-lived people
Centennial centenarians are considered the best human model for studying the biological determinants of longevity, which have reached the most extreme values of human life expectancy (45).
Several studies compared circulating insulin and IGF-1 levels in long-livers with those in younger controls (46).
Metabolic age-related remodeling is a physiological process that occurs in the entire population. Aging is often associated with a decrease in glucose tolerance, which is associated with increased insulin resistance (47), but long-lived people have an exception. It was found (48) that insulin resistance increases with age and decreases in people older than 90 years living in southern Italy. Indeed, long-lived subjects showed higher insulin sensitivity and better beta-cell function than younger subjects. This difference also did not depend on the main anthropometric and metabolic factors. In 100-year-old patients, the plasma glucose concentration for 2 hours was lower than in the elderly (average age 78 years). In long-livers, insulin-mediated glucose intake was higher than in elderly controls during euglycemic glucose uptake, which maintained the preserved glucose tolerance and insulin action in this long-living group (49, 50). Similar results confirming the best sensitivity to insulin were observed in other populations of long-livers (51, 52).
In addition, long-livers showed the preserved effect of insulin not only on glucose metabolism, but also on adipose tissue. In fact, insulin infusion is usually associated with inhibition of lipolysis and, thus, with a significant decrease in the concentration of free fatty acids and triglycerides in plasma. In long-livers, the inhibitory activity of insulin during lipolysis was higher than in the control participants (mean age 78 years) (50). It should be noted that in long-livers, in comparison with adult controls, lower sympathetic tone is also observed, which may be associated with a better action of insulin and, therefore, with a low level of fasting insulin in plasma (53, 54).
The data on the IGF-1 system regarding life expectancy are still contradictory in long-lived subjects (46). Paolisso et al. (55) described an increased plasma IGF-1 / IGFBP-3 ratio in healthy long-livers compared with older people. They suggested that this increased ratio indicates a higher bioavailability of IGF-1, which contributes to the improvement of insulin action in long-livers. Bonafè et al. (56) reported that subjects with at least the A allele of the IGF-1 receptor gene (G / A, codon 1013) had low plasma levels of free IGF-1 and were more prevalent among long-lived individuals. Arai et al. (57) described relatively low levels of serum IGF-1 in the Japanese long-livers population. In this population, the lowest rates of both IGF-1 and IGFBP-3 were associated with increased mortality (58).
These conflicting results probably reflect the complexity of the IGF system and ethnic differences in the registered population. In addition, centenarians were often compared with a control group of younger subjects. Thus, in most of these studies, it was impossible to conclude whether the differences between IGF-1 in both groups were associated with different life expectancy or reflected a physiologically age-dependent decrease in IGF-1. Indeed, there are several limitations to the study of long-livers: (1) low prevalence (1 centenary per 5-10,000 inhabitants), (2) weakness due to extreme age (almost 95% of long-livers have at least 1 asthenia criterion), (3 ) the absence of a control group of the same age (45, 59). Due to these limitations, this human model is not suitable for studying age-related variables,
The descendants of centenarians are another interesting model for determining the relevant factors associated with human longevity and healthy aging. A consistent set of observations in different countries suggests that the descendants of long-livers are healthier than representatives of the same demographic groups (51, 60, 61) and biologically (epigenetically) younger than their chronological age (62). In general, these studies show that relatives of centenarians are more likely to live longer and have good health (60, 63). In addition, the study of the offspring of long-livers has the advantage of having an appropriate demographically selected control group consisting of a progeny of comparable age, in which both parents were born in the same cohort of centenarians, but died before the threshold age. after which people are classified as “centenarians.” This strategy is critical to preventing cohort effects. Thus, the model of offspring of long-livers can overcome some of the limitations that are found in the study of long-livers (rarity, weakness, and lack of proper control) (60).
In several studies, the IGF-1 / insulin system was characterized in descendants of long-livers and in the corresponding control group.
We evaluated the circulating bioactivity of IGF-1, measured using an innovative kinase receptor activation assay (KIRA) performed on long-livers, long-livers descendants, and comparable control offspring. The offspring of long-livers and long-livers had a relatively lower circulating biological activity of IGF-1 compared to the control group. Interestingly, the biological activity of IGF-1 in descendants of long-livers was inversely related to insulin sensitivity (51).
Suh et al. ( 64 ) оценивали уровни IGF-1 в сыворотке у потомков еврейских долгожителей ашкенази и в сопостаимых по возрасту контролях. У детей долгожителей женского пола уровень IGF-1 в сыворотке был на 35% выше, чем у контрольних участников. Эта разница может представлять компенсаторный ответ на снижение передачи сигналов рецептора IGF-1. Кроме того, у еврейских долгожителей ашкенази было описано чрезмерное присутствие гетерозиготных мутаций в гене рецептора IGF-1 вместе с относительно высокими уровнями IGF-1 в сыворотке и ослабленной активностью рецептора IGF-1 по сравнению с контрольной группой без семейного долголетия.
To study longevity, other authors examined ninety-year-old brothers and sisters and their descendants. In the Leyden study of longevity, 421 families were selected, consisting of at least two reporters, brothers and sisters, their descendants and partners of descendants as a control. In these populations, serum glucose, insulin, and triglycerides were the best biomarker for healthy aging (low glucose and insulin levels were considered healthy) (65). Nine-year-old centenarians in the lowest IGF-1 / IGFBP-3 ratio in the bloodstream were associated with better survival (66). Descendants of nine-year-olds showed better insulin sensitivity compared to their partners, while similar levels of fasting serum IGF-1 and IGFBP-3 were observed in both fasting groups (67). Interesting,
Another approach taken to study longevity in humans is to select family components for exceptional longevity and healthy aging based on strict criteria, such as the Long Life Family Study, a measure of family life span. These families, selected for exceptional life expectancy, were compared with a control group without a family history of longevity (69). In this population, circulating levels of IGF-1 proved to be a reliable age-related biomarker (70).
In support of the potential role of the GH / IGF-1 / insulin system in human longevity, there are many genetic studies. Indeed, it has been found that several genetic loci are associated with circulating levels of IGF-1 and IGFBP-3 and are potentially capable of affecting aging (71). A genome-wide analysis of associations conducted in ninety-year-olds and patient populations aged <60 years showed a clear link between the genetic variation of genes involved in the insulin / IGF-1 pathway and human lifespan (72). In a prospective study of older people, women with a genetic profile, indicating a decrease in insulin / IGF-1 signaling activity, showed longer life spans (73). In four independent cohorts of long-lived individuals, a linear increase in the prevalence of exogenous 3 (G3R) GH receptor homozygosity with age has recently been described. The presence of the d3 / d3 genotype increased life expectancy by about 10 years (74).
IGF-1 system and calorie restriction
One of the most convincing observations in the biology of aging is the ability to limit calories to prevent or delay some age-related diseases and increase the life expectancy of mammals (75 - 78). The biological mechanisms of this phenomenon are not entirely clear, but the suggestion was made about the potential involvement of corresponding changes in energy metabolism, the endocrine system and oxidative damage.
Calorie restriction causes numerous hormonal changes. In rodents, caloric restriction without starvation suppressed circulating levels of IGF-1 and insulin in proportion to the level of restriction, increased insulin sensitivity and resistance to stress and toxicity, and reduced the risk of cancer (79, 80). Interestingly, most of these characteristics observed in wild-type mice during caloric restriction resemble those reported in mice that are long-living due to genetic impairment of GH / IGF-1 / insulin signaling, as described previously.
Randomized clinical trials in humans have shown that calorie restriction does not reduce serum IGF-1 unless protein intake is reduced (81, 82). However, a recent meta-analysis evaluating the effect of nutritional restriction on the recognized biomarkers of healthy aging has shown a decrease in human IGF-1 levels in blood (83).
Other hormonal changes, such as a decrease in insulin levels, thyroid hormones and leptin levels, as well as an increase in adiponectin and insulin sensitivity, were observed during diet restriction (85, 86).
This hormonal adaptation can play an important role in prolonging life through several mechanisms:
1) Decreased metabolic rate, cell proliferation and oxidative stress. In fact, IGF-1 is a potent growth factor, and thyroid hormone is a potent stimulator of metabolic rate and metabolic rate. In addition, transcription patterns suggest that chronic moderate calorie restriction in adults slows down the aging process, switching cell metabolism from growth to maintenance and recovery (84).
2) Reducing the accumulation of aging cells. Cell aging has been shown to be a key mediator of aging (87). Over time, protein homeostasis decreases and damage accumulates. Interestingly, some age-related diseases can be delayed by weakening the accumulation of aging cells (88, 89). Typically, the mTOR pathway is activated by several signals, including nutrients, IGF-1, and insulin (Fig. 2). The deregulation of this pathway, which was reported after calorie restriction, increased the lifespan of some organisms. This effect, apparently, is secondary to an increase in autophagy, a cytoprotective digestion process. In fact, autophagy is a cell processing process that can remove old or damaged cellular components, preventing the accumulation of aging cells (90,
3) Counteracting inflammation. Dietary intervention in both animals and humans can slow the aging process, weakening the inflammatory status of a weak degree (83, 92). The mechanisms underlying the anti-inflammatory activity of nutritional restriction are not clearly defined. It is believed that this effect is due to a decrease in fat mass and proinflammatory adipokines, as well as an improvement in the integrity of the intestinal barrier observed during dietary intervention (93, 94).
Интересно отметить, что эндокринный биохимический профиль, наблюдаемый у субъектов во время ограничения калорийности, сопоставим с таковым у долгожителей, что подтверждает потенциальную роль эндокринной системы в модуляции продолжительности жизни. В дополнение к увеличению чувствительности к инсулину и снижению уровней IGF-1 в плазме / сыворотке, в нескольких исследованиях было показано повышение уровня циркулирующего адипонектина и снижение уровней циркулирующих лептина и тиреоидных гормонов у долгоживущих людей по сравнению с более молодыми субъектами.
Adipose tissue is an endocrine organ that produces several cytokines involved in relevant processes, such as energy metabolism, lipid and glucose homeostasis, and modulation of the inflammatory response. Visceral adipose tissue plays a major role in the development of metabolic diseases (95). Aging is associated with an increase in fat mass and redistribution of adipose tissue, characterized by a loss of peripheral subcutaneous fat and an accumulation of visceral fat. In older people, changes in the secretion, synthesis and function of adipokines have been described, probably due to an imbalance in the function, proliferation, size and number of fat cells (86). Adiponectin is an insulin-sensitizing, anti-inflammatory and anti-atherogenic cytokine. Adiponectin circulates in the blood in several forms: trimer, hexamer, high molecular weight multimer (HMW) and globular adiponectin (proteolytically cleaved form). The multimer HMW is believed to be a more active form of adiponectin for protection against insulin resistance and diabetes (96). Circulating adiponectin is independently and negatively associated with aspects of the metabolic syndrome, including insulin resistance, body weight, blood pressure and serum lipids. Leptin is mainly produced in the subcutaneous and to a lesser extent in the visceral white adipose tissue. This cytokine regulates food intake, energy expenditure, and atherogenesis. Leptin promotes weight loss by reducing appetite and stimulating metabolism and has pro-inflammatory properties (97). that the multimer HMW is a more active form of adiponectin in protection against insulin resistance and diabetes (96). Circulating adiponectin is independently and negatively associated with aspects of the metabolic syndrome, including insulin resistance, body weight, blood pressure and serum lipids. Leptin is mainly produced in the subcutaneous and to a lesser extent in the visceral white adipose tissue. This cytokine regulates food intake, energy expenditure, and atherogenesis. Leptin promotes weight loss by reducing appetite and stimulating metabolism and has pro-inflammatory properties (97). that the multimer HMW is a more active form of adiponectin in protection against insulin resistance and diabetes (96). Circulating adiponectin is independently and negatively associated with aspects of the metabolic syndrome, including insulin resistance, body weight, blood pressure and serum lipids. Leptin is mainly produced in the subcutaneous and to a lesser extent in the visceral white adipose tissue. This cytokine regulates food intake, energy expenditure, and atherogenesis. Leptin promotes weight loss by reducing appetite and stimulating metabolism and has pro-inflammatory properties (97). blood pressure and serum lipids. Leptin is mainly produced in the subcutaneous and to a lesser extent in the visceral white adipose tissue. This cytokine regulates food intake, energy expenditure, and atherogenesis. Leptin promotes weight loss by reducing appetite and stimulating metabolism and has pro-inflammatory properties (97). blood pressure and serum lipids. Leptin is mainly produced in the subcutaneous and to a lesser extent in the visceral white adipose tissue. This cytokine regulates food intake, energy expenditure, and atherogenesis. Leptin promotes weight loss by reducing appetite and stimulating metabolism and has pro-inflammatory properties (97).
Several studies have reported that long-livers have higher plasma adiponectin levels and lower leptin concentrations than younger controls (53, 98 - 102). All forms of adiponectin were significantly increased in long-livers, but the multimer HMW was significantly higher (99). In long-livers, high adiponectin concentrations turned out to be independent of BMI, renal or cardiovascular function and were associated with a favorable metabolic phenotype (higher levels of HDL-C, lower glycated hemoglobin, insulin, HOMA-IR and triglycerides) (98, 99). Elevated adiponectin levels have also been found in offspring of long-lived subjects (over 95 years old) (103).
A decrease in the level of thyroid hormones is apparently characteristic of long-livers. Mariotti et al. (104) reported that healthy centenarians had lower serum TSH and FT3 levels and higher serum rT3 levels compared to those observed in other control groups. In another Italian population of long-livers, the total T4 values were below the normal range in 60% of the examined (105). Baranovskaya et al. reported that serum T3 levels in centenarians were lower than those in early elderly and young women (52). We recently characterized the thyroid function profile in an Italian cohort of 672 patients (aged 52–113 years). An age-related decrease in FT3 level and FT3 / FT4 ratios were noted, while FT4 and TSH increase with age (106).
Корсонелло и соавт. ( 108 ) обнаружили у родственников долгожителей (потомство или племянницы / племянники) более низкие сопутствующие заболевания, уровни FT3, FT4 и TSH, чем у сопоставимых по возрасту контролей, которые не были родственниками долгожителей. В другой итальянской популяции более низкий уровень FT4 в плазме наблюдался у потомков долгожителей по сравнению с контрольной группой того же возраста ( 60 ).
In general, long-livers are thin (109) and adhere to healthy eating habits (110). Similar to subjects during caloric restriction, long-livers observed slower cell growth / metabolism, better control of signal transmission and increased autophagy. Through analysis of DNA methylation throughout the genome in centenarians and their descendants, we have identified epigenetically modulated genes and pathways that are potentially involved in aging and longevity. Our results indicate that these populations were characterized by better conservation of DNA methylation status, slower cell growth / metabolism and better control of signal transmission through epigenetic mechanisms (111). In long-livers, bioenergetic function is preserved due to mitochondrial hypertrophy, which can compensate for functional defects (112). In addition, healthy long-livers have high levels of autophagy, as evidenced by higher serum beclin-1 levels in comparison with both young patients with myocardial infarction and healthy controls (113). An increase in autophagic activity was also observed in subjects belonging to families with exceptional longevity (114).
The corresponding phenomenon arises in relation to the inflammatory status, which weakens in subjects after calorie restriction (115, 116) and is high in long-livers (117 - 119). With aging, there is a state of mild and chronic inflammatory pathology (age-related inflammation) and an increased prevalence of a number of diseases, such as cardiovascular diseases, atherosclerosis, tumors, cognitive impairment, osteoarthritis and diabetes (120, 121). Therefore, the weakening of chronic inflammatory status after calorie restriction is a beneficial effect. Centennial centenarians show signs of inflammation, but at the same time, it seems, spared its harmful effects. This obvious paradox can be explained by the fact
These data suggest the general mechanisms for increasing longevity and delaying age-related diseases found in long-livers and mammals during a calorie-restricted diet.
Opinion of the authors.
Preclinical models have provided a deeper insight into the aging process using consistent data that takes into account the role of the GH / IGF-1 / insulin system in modulating life expectancy. Although it is well known that increased insulin sensitivity and low insulin levels are associated with improved survival, there is some evidence showing that a weakening of the GH / IGF-1 axis can have a beneficial effect on increasing life expectancy in humans. However, it is still unknown what are the optimal levels of IGF-1 throughout life to live longer and be healthier. In addition, IGF-1 receptor sensitivity and activation of the post-receptor pathway have not been evaluated in most studies involving long-lived subjects. Consequently, it is not possible to determine the actual state of IGF-1 receptor signaling activation by a simple dose of circulating levels of IGF-1. This makes it difficult to identify pharmacological or environmental strategies that target this system to increase longevity and promote healthy aging. A comprehensive understanding of these aspects remains the main problem for identifying interventions aimed at slowing down human aging and for use in rehabilitation medicine. Future studies should evaluate the functional state of IGF-1 receptor signaling, including through transcriptional profiling and analysis of functional networks regarding IGF-1 regulated genes in long-lived subjects. This makes it difficult to identify pharmacological or environmental strategies that target this system to increase longevity and promote healthy aging. A comprehensive understanding of these aspects remains the main problem for identifying interventions aimed at slowing down human aging and for use in rehabilitation medicine. Future studies should evaluate the functional state of IGF-1 receptor signaling, including through transcriptional profiling and analysis of functional networks regarding IGF-1 regulated genes in long-lived subjects. This makes it difficult to identify pharmacological or environmental strategies that target this system to increase longevity and promote healthy aging. A comprehensive understanding of these aspects remains the main problem for identifying interventions aimed at slowing down human aging and for use in rehabilitation medicine. Future studies should evaluate the functional state of IGF-1 receptor signaling, including through transcriptional profiling and analysis of functional networks regarding IGF-1 regulated genes in long-lived subjects. and applications in rehabilitation medicine. Future studies should evaluate the functional state of IGF-1 receptor signaling, including through transcriptional profiling and analysis of functional networks regarding IGF-1 regulated genes in long-lived subjects. and applications in rehabilitation medicine. Future studies should evaluate the functional state of IGF-1 receptor signaling, including through transcriptional profiling and analysis of functional networks regarding IGF-1 regulated genes in long-lived subjects.
Conclusions A
striking similarity has been described with respect to the endocrine profile between long-livers and subjects after a calorie-restricted diet. The endocrine and metabolic adaptation observed in both models can be a physiological strategy for increasing life expectancy due to slower cell growth / metabolism, slower loss of the natural physiological reserve, the transition of cellular metabolism from cell proliferation to regenerative activity, and reducing the accumulation of aging cells. Apparently, these mechanisms, at least partially, are mediated by modulation of the GH / IGF-1 / insulin system.
List of references
- Franceschi C, Valensin S, Bonafè M, Paolisso G, Yashin AI, Monti D, et al .. The network and the remodeling theories of aging: historical background and new perspectives. Exp Gerontol. (2000) 35: 879–96. 10.1016 / S0531-5565 (00) 00172-8.
- Bartke A, Darcy J. GH and ageing: Pitfalls and new insights. Best Pract Res Clin Endocrinol Metab. (2017) 31: 113–25. 10.1016 / j.beem.2017.02.005
- Vitale G, Salvioli S, Franceschi C. Oxidative stress and the aging endocrine system. Nat Rev Endocrinol. (2013) 9: 228–40. 10.1038 / nrendo.2013.29
- Vitale G, Cesari M, Mari D. Aging of the endocrine system and its potential impact on sarcopenia. Eur J Intern Med. (2016) 35: 10-15. 10.1016 / j.ejim.2016.07.017
- Reddy SSK, Chaiban JT. The Endocrinology of aging: a key to longevity “Great Expectations”. Endocr Pract. (2017) 23: 1107–16. 10.4158 / EP171793.RA
- Junnila RK, List EO, Berryman DE, Murrey JW, Kopchick JJ. The GH / IGF-1 axis in ageing and longevity. Nat Rev Endocrinol. (2013) 9: 366–76. 10.1038 / nrendo.2013.67
- Yakar S, Adamo ML. Insulin-like growth factor 1 physiology: lessons from mouse models. Endocrinol Metab Clin North Am. (2012) 41: 231–47. 10.1016 / j.ecl.2012.04.008 [PMC free article] [PubMed] [CrossRef] [Google Scholar]
- Higashi Y, Sukhanov S, Anwar A, Shai SY, Delafontaine P. IGF-1, oxidative stress and atheroprotection. Trends Endocrinol Metab. (2010) 21: 245-54. 10.1016 / j.tem.2009.12.005 [PMC free article] [PubMed] [CrossRef] [Google Scholar]
- Belfiore A, Malaguarnera R, Vella V, Lawrence MC, Sciacca L, Frasca F, et al .. Insulin receptor isoforms in physiology and disease: an updated view. Endocr rev. (2017) 38: 379-431. 10.1210 / er.2017-00073 [PMC free article] [PubMed] [CrossRef] [Google Scholar]
- Kenyon C, Chang J, Gensch E, Rudner A, Tabtiang RAC ... elegans mutant that lives twice as long as wild type. Nature (1993) 366: 461-4. 10.1038 / 366461a0 [PubMed] [CrossRef] [Google Scholar]
- Kimura KD, Tissenbaum HA, Liu Y, Ruvkun G. Daf-2, an insulin receptor-like gene that regulates longevity and diapause in Caenorhabditis elegans. Science (1997) 277: 942-6. 10.1126 / science.277.5328.942 [PubMed] [CrossRef] [Google Scholar]
- Tatar M, Kopelman A, Epstein D, Tu MP, Yin CM, Garofalo RS, et al .. A mutant Drosophila insulin receptor homolog that extends life-span and impairs neuroendocrine function. Science (2001) 292: 107-10. 10.1126 / science.1057987 [PubMed] [CrossRef] [Google Scholar]
- Clancy DJ, Gems D, Harshman LG, Oldham S, Stocker H, Hafen E, et al .. Extension of life-span by loss of CHICO, a Drosophila insulin receptor substrate protein. Science (2001) 292: 104-6. 10.1126 / science.1057991 [PubMed] [CrossRef] [Google Scholar]
- Libina N, Berman JR, Kenyon C. Tissue-specific activities of C. elegans DAF-16 in the regulation of lifespan. Cell (2003) 115: 489-502. 10.1016 / S0092-8674 (03) 00889-4 [PubMed] [CrossRef] [Google Scholar]
- Broughton S, Partridge L. Insulin / IGF-like signalling, the central nervous system and aging. Biochem J. (2009) 418: 1-12. 10.1042 / BJ20082102 [PubMed] [CrossRef] [Google Scholar]
- Reindl KM, Sheridan MA. Peripheral regulation of the growth hormone-insulin-like growth factor system in fish and other vertebrates. Comp Biochem Physiol A Mol Integr Physiol. (2012) 163: 231–45. 10.1016 / j.cbpa.2012/08/08.003 [PubMed] [CrossRef] [Google Scholar]
- Snell GD. Dwarf, a new mendelian recessive character of the house mouse. Proc Natl Acad Sci USA. (1929) 15: 733–4. 10.1073 / pnas.15.9.733 [PMC free article] [PubMed] [CrossRef] [Google Scholar]
- Berryman D, Christiansen JS, Johannsson G, Thorner MO, Kopchick JJ. Role of the GH / IGF-1 axis in lifespan and healthspan: lessons from animal models. Growth Horm IGF Res. (2008) 18: 455–71. 10.1016 / j.ghir.2008.05.005 [PMC free article] [PubMed] [CrossRef] [Google Scholar]
- Flurkey K, Papaconstantinou J, Miller RA, Harrison DE. Lifespan extension and delayed immune and collagen aging in mutant mice with defects in growth hormone production. Proc Natl Acad Sci USA. (2001) 98: 6736–41. 10.1073 / pnas.111158898 [PMC free article] [PubMed] [CrossRef] [Google Scholar]
- Brown-Borg HM, Borg KE, Meliska CJ, Bartke A. Dwarf mice and the aging process. Nature (1996) 384: 33. 10.1038 / 384033a0 [PubMed] [CrossRef] [Google Scholar]
- Bartke A, Wright JC, Mattison JA, Ingram DK, Miller RA, Roth GS. Extending the lifespan of long-lived mice. Nature (2001) 414: 412. 10.1038 / 35106646 [PubMed] [CrossRef] [Google Scholar]
- Panici JA, Harper JM, Miller RA, Bartke A, Spong A, Masternak MM. Early life growth hormone treatment shortens longevity and decreases cellular stress resistance in long-lived mutant mice. FASEB J. (2010) 24: 5073–9. 10.1096 / fj.10-163253 [PMC free article] [PubMed] [CrossRef] [Google Scholar]
- Sun LY, Spong A, Swindell WR, Fang Y, Hill C, Huber JA, et al .. Growth hormone-releasing hormone disruption extends lifespan and regulates response to caloric restriction in mice. Elife (2013) 2: e01098. 10.7554 / eLife.01098 [PMC free article] [PubMed] [CrossRef] [Google Scholar]
- Coschigano KT, Clemmons D, Bellush LL, Kopchick JJ. Assessment of growth parameters and life span of GHR / BP gene-disrupted mice. Endocrinology (2000) 141: 2608-13. 10.1210 / endo.141.7.7586 [PubMed] [CrossRef] [Google Scholar]
- Ikeno Y, Hubbard GB, Lee S, Cortez LA, Lew CM, Webb CR, et al .. Reduced incidence and delayed occurrence of fatal neoplastic diseases in growth hormone receptor / binding protein knockout mice. J Gerontol A Biol Sci Med Sci. (2009) 64: 522–9. 10.1093 / gerona / glp017 [PMC free article] [PubMed] [CrossRef] [Google Scholar]
- Gesing A, Wiesenborn D, Do A, Menon V, Schneider A, Victoria B, et al .. A long-lived mouse lacking both growth hormone and growth hormone receptor: a new animal model for aging studies. J Gerontol A Biol Sci Med Sci. (2017) 72: 1054–61. 10.1093 / gerona / glw193 [PMC free article] [PubMed] [CrossRef] [Google Scholar]
- Bonkowski MS, Rocha JS, Masternak MM, Al Regaiey KA, Bartke A. Targeted disruption of growth hormone receptor interferes with the beneficial actions of calorie restriction. Proc Natl Acad Sci USA. (2006) 103: 7901-5. 10.1073 / pnas.0600161103 [PMC free article] [PubMed] [CrossRef] [Google Scholar]
- Coschigano KT, Holland AN, Riders ME, List EO, Flyvbjerg A, Kopchick JJ. Deletion, but not antagonism, of the mouse growth hormone receptor results in severely reduced body weights, insulin, and insulin-like growth factor I levels and increased life span. Endocrinology (2003) 144: 3799-810. 10.1210 / en.2003-0374 [PubMed] [CrossRef] [Google Scholar]
- Holzenberger M, Dupont J, Ducos B, Leneuve P, Géloën A, Even PC, et al .. IGF-1 receptor regulates lifespan and resistance to oxidative stress in mice. Nature (2003) 421: 182–7. 10.1038 / nature01298 [PubMed] [CrossRef] [Google Scholar]
- Bokov AF, Garg N, Ikeno Y, Thakur S, Musi N, DeFronzo RA, et al .. Does reduced IGF-1R signaling in Igf1r +/− mice alter aging? Plos ONE (2011) 6: e26891. 10.1371 / journal.pone.0026891 [PMC free article] [PubMed] [CrossRef] [Google Scholar]
- Xu J, Gontier G, Chaker Z, Lacube P, Dupont J, Holzenberger M. Longevity effect of IGF-1R +/− mutation depends on genetic background-specific receptor activation. Aging Cell (2014) 13: 19–28. 10.1111 / acel.12145 [PMC free article] [PubMed] [CrossRef] [Google Scholar]
- Mulvey L, Sinclair A, Selman C. Lifespan modulation in mice and the confounding effects of genetic background. J Genet Genomics (2014) 41: 497-503. 10.1016 / j.jgg.2014.06.002 [PMC free article] [PubMed] [CrossRef] [Google Scholar]
- Kappeler L, De Magalhaes Filho C, Dupont J, Leneuve P, Cervera P, Périn L, et al .. Brain IGF-1 receptors control mammalian growth and lifespan through a neuroendocrine mechanism. PLOS Biol. (2008) 6: e254. 10.1371 / journal.pbio.0060254tt [PMC free article] [PubMed] [CrossRef] [Google Scholar]
- Svensson J, Sjögren K, Fäldt J, Andersson N, Isaksson O, Jansson JO, et al. Liver-derived IGF-1 regulates mean life span in mice. PLoS ONE (2011) 6: e22640 10.1371 / journal.pone.0022640 [PMC free article] [PubMed] [CrossRef] [Google Scholar]
- Conover CA, Bale LK. Loss of pregnancy-associated plasma protein A extends lifespan in mice. Aging Cell (2007) 6: 727–9. 10.1111 / j.1474-9726.2007.00328.x [PubMed] [CrossRef] [Google Scholar]
- Conover CA. Role of PAPP-A in aging and age-related disease. Exp Gerontol. (2013) 48: 612-3. 10.1016 / j.exger.2012.06.017 [PMC free article] [PubMed] [CrossRef] [Google Scholar]
- Selman C, Lingard S, Choudhury AI, Batterham RL, Claret M, Clements M, et al .. Evidence for lifespan extension and delayed age-related biomarkers in insulin receptor substrate 1 null mice. FASEB J. (2008) 22: 807-18. 10.1096 / fj.07-9261com [PubMed] [CrossRef] [Google Scholar]
- Taguchi A, Wartschow LM, White MF. Brain IRS2 signaling coordinates life span and nutrient homeostasis. Science (2000) 317: 369–72. 10.1126 / science.1142179 [PubMed] [CrossRef] [Google Scholar]
- Kuro M, Matsumura Y, Aizawa H, Kawaguchi H, Suga T, Utsugu T, et al. Mutation of the mouse Klotho gene leads to a syndrome resembling ageing. Nature (1997) 390: 45-51. 10.1038 / 36285 [PubMed] [CrossRef] [Google Scholar]
- Kurosu H, Yamamoto M, Clark JD, Pastor JV, Nandi A, Gurnani P, et al .. Suppression of aging in mice by the hormone Klotho. Science (2005) 309: 1829–33. 10.1126 / science.1112766 [PMC free article] [PubMed] [CrossRef] [Google Scholar]
- Migliaccio E, Giorgio M, Mele S, Pelicci G, Reboldi P, Pandolfi PP, et al. The p66 Shc adapter protein controls oxidative stress response and life span in mammals. Nature (1999) 402: 309–13. 10.1038 / 46311 [PubMed] [CrossRef] [Google Scholar]
- Ramsey JJ, Tran D, Giorgio M, Griffey SM, Koehne A, Laing ST, et al .. The influence of Shc proteins on life span in mice. J Gerontol A Biol Sci Med Sci. (2014) 69: 1177–85. 10.1093 / gerona / glt198 [PMC free article] [PubMed] [CrossRef] [Google Scholar]
- Ashpole NM, Logan S, Yabluchanskiy A, Mitschelen MC, Yan H, Farley JA, et al .. IGF-1 has sexually dimorphic, pleiotropic, and time-dependent effects on healthspan, pathology, and lifespan. Geroscience (2017) 39: 129–45. 10.1007 / s11357-017-9971-0 [PMC free article] [PubMed] [CrossRef] [Google Scholar]
- Mao K, Quipildor GF, Tabrizian T, Novaj A, Guan F, Walters RO, et al .. Late-life targeting of the IGF-1 receptor improves healthspan and lifespan in female mice. Nat Commun. (2018) 9: 2394. 10.1038 / s41467-018-04805-5 [PMC free article] [PubMed] [CrossRef] [Google Scholar]
- Franceschi C, Passarino G, Mari D, Monti D. Centenarians as a 21st century healthy aging model: a legacy of humanity and the need for a world-wide consortium (WWC100 +). Mech Ageing Dev. (2017) 165 (Pt. B): 55–8. 10.1016 / j.mad.2017.06.002 [PubMed] [CrossRef] [Google Scholar]
- Vitale G, Barbieri M, Kamenetskaya M, Paolisso G. GH / IGF-I / insulin system in centenarians. Mech Ageing Dev. (2017) 165: 107–114. 10.1016 / j.mad.2016.12.001 [PubMed] [CrossRef] [Google Scholar]
- Ferrannini E, Vichi S, Beck-Nielsen H, Laasko M, Paolisso G, Smith U. For European Group for the Study of Insulin Resistance (EGIR). Insulin action and age. Diabetes (1996) 45: 947-53. 10.2337 / diab.45.7.947 [PubMed] [CrossRef] [Google Scholar]
- Paolisso G, Barbieri M, Rizzo MR, Carella C, Rotondi M, Bonafè M, et al. Low insulin resistance and preserved beta-cell function contribute to human longevity but are not associated with TH-INS genes. Exp Gerontol. (2001) 37: 149–56. 10.1016 / S0531-5565 (01) 00148-6 [PubMed] [CrossRef] [Google Scholar]
- Paolisso G, Gambardella A, Ammendola S, D'Amore A, Balbi V, Varricchio M, et al .. Glucose tolerance and insulin action in healty centenarians. Am J Physiol. (1996) 270: E890-4. 10.1152 / ajpendo.1996.270.5.E890 [PubMed] [CrossRef] [Google Scholar]
- Paolisso G, Gambardella A, Ammendola S, Tagliamonte MR, Rizzo MR, Capurso A, et al .. Preserved antilipolytic insulin action is associated with a less atherogenic plasma lipid profile in healthy centenarians. J Am Geriatr Soc. (1997) 45: 1504–9. 10.1111 / j.1532-5415.1997.tb03203.x [PubMed] [CrossRef] [Google Scholar]
- Vitale G, Brugts M, Ogliari G, Castaldi D, Fatti L, Varewijck A, et al .. Low circulating IGF-I bioactivity is associated with human longevity: findings in centenarians' offspring. Aging (2012) 4: 580–89. 10.18632 / aging.100484 [PMC free article] [PubMed] [CrossRef] [Google Scholar]
- Baranowska B, Wolinska-Witort E, Bik W, Baranowska-Bik A, Martynska L, Broczek K, et al .. Evaluation of neuroendocrine status in longevity. Neurobiol Aging (2007) 28: 774–83. 10.1016 / j.neurobiolaging.2006.03.014 [PubMed] [CrossRef] [Google Scholar]
- Paolisso G, Manzella D, Barbieri M, Rizzo MR, Gambardella A, Varricchio M. Baseline heart rate variability in healthy centenarians: differences vs. aged subject. Clin. Sci. (1999) 97: 579–84. 10.1042 / cs0970579 [PubMed] [CrossRef] [Google Scholar]
- Paolisso G, Tagliamonte MR, Rizzo MR, Carella C, Gambardella A, Barbieri M, et al. Low plasma Insulin like growth factor-1 concentration predict worsening of insulin mediated glucose uptake in the elderly. J. Am. Geriatr. Soc. (1999) 47: 1312–8. 10.1111 / j.1532-5415.1999.tb07431.x [PubMed] [CrossRef] [Google Scholar]
- Paolisso G, Ammendola S, Del Buono A, Gambardella A, Riondino M, Tagliamonte MR, et al .. Serum levels of insulin-like growth factor-I (IGF-I) and IGF-binding protein-3 in healthy centenarians: relationship with plasma leptin and lipid concentrations, insulin action, and cognitive function. J Clin Endocrinol Metab. (1997) 82: 2204–9. 10.1210 / jcem.82.7.4087 [PubMed] [CrossRef] [Google Scholar]
- Bonafè M, Barbieri M, Marchegiani F, Olivieri F, Ragno E, Giampieri C, et al .. Polymorphic variants of insulin-like growth factor I (IGF-I) receptor and phosphoinositide 3-kinase genes affect IGF-I plasma levels and human longevity: cues for an evolutionarily conserved mechanism of life span control. J Clin Endocrinol Metab. (2003) 88: 3299-304. 10.1210 / jc.2002-021810 [PubMed] [CrossRef] [Google Scholar]
- Arai Y, Hirose N, Yamamura K, Shimizu K, Takayama M, Ebihara Y, et al .. Serum insulin-like growth factor-1 in centenarians: implications of IGF-1 as a rapid turnover protein. J Gerontol A Biol Sci Med Sci. (2001) 56: M79–82. 10.1093 / gerona / 56.2.M79 [PubMed] [CrossRef] [Google Scholar]
- Arai Y, Takayama M, Gondo Y, Inagaki H, Yamamura K, Nakazawa S, et al .. Adipose endocrine function, insulin-like growth factor-1 axis, and exceptional survival beyond 100 years of age. J Gerontol A Biol Sci Med Sci. (2008) 63: 1209-18. 10.1093 / gerona / 63.11.1209 [PubMed] [CrossRef] [Google Scholar]
- Herr M, Jeune B, Fors S, Andersen-Ranberg K, Ankri J, Arai Y, et al .. Frailty and associated factors among centenarians in the 5-COOP countries. Gerontology (2018) 64: 521–31. 10.1159 / 000489955 [PubMed] [CrossRef] [Google Scholar]
- Bucci L, Ostan R, Cevenini E, Pini E, Scurti M, Vitale G, et al .. Centenarians' offspring as a model of healthy aging: a reappraisal of the data on Italian subjects and a comprehensive overview. Aging (Albany. NY). (2016) 8: 1–11. 10.18632 / aging.100912 [PMC free article] [PubMed] [CrossRef] [Google Scholar]
- Gueresi P, Miglio R, Monti D, Mari D, Sansoni P, Caruso C, et al .. Does the longevity of one or both parents influence the health status of their offspring? Exp Gerontol. (2013) 48: 395-400. 10.1016 / j.exger.2013.02.004 [PubMed] [CrossRef] [Google Scholar]
- Horvath S, Pirazzini C, Bacalini MG, Gentilini D, Di Blasio AM, Delledonne M, et al .. Decreased epigenetic age of PBMCs from Italian semi-supercentenarians and their offspring. Aging (2015) 7: 1159–70. 10.18632 / aging.100861 [PMC free article] [PubMed] [CrossRef] [Google Scholar]
- Caselli G, Pozzi L, Vaupel JW, Deiana L, Pes G, Carru C, et al .. Family clustering in Sardinian longevity: a genealogical approach. Exp Gerontol. (2006) 41: 727–36. 10.1016 / j.exger.2006.05.009 [PubMed] [CrossRef] [Google Scholar]
- Suh Y, Atzmon G, Cho MO, Hwang D, Liu B, Leahy DJ, et al. Functionally significant insulin-like growth factor I receptor mutations in centenarians. Proc Natl Acad Sci USA. (2008) 105: 3438–42. 10.1073 / pnas.0705467105 [PMC free article] [PubMed] [CrossRef] [Google Scholar]
- Deelen J, van den Akker EB, Trompet S, van Heemst D, Mooijaart SP, Slagboom PE, Beekman M. Employing biomarkers of healthy aging for leveraging genetic studies into human longevity. Exp Gerontol. (2016) 82: 166–74. 10.1016 / j.exger.2016.06.01.01 [PubMed] [CrossRef] [Google Scholar]
- van der Spoel E, Rozing MP, Houwing-Duistermaat JJ, Slagboom PE, Beekman M, de Craen AJ, et al .. Association analysis of insulin-like growth factor-1 axis parameters with survival and functional status in nonagenarians of the Leiden Longevity Study. Aging (2015) 7: 956–63. 10.18632 / aging.100841 [PMC free article] [PubMed] [CrossRef] [Google Scholar]
- Rozing MP, Westendorp RG, Frölich M, de Craen AJ, Beekman M, Heijmans BT, et al .. Human insulin / IGF-1 and familial longevity at middle age. Aging (2009) 1: 714–22. 10.18632 / aging.100071 [PMC free article] [PubMed] [CrossRef] [Google Scholar]
- van der Spoel E, Jansen SW, Akintola AA, Ballieux BE, Cobbaert CM, Slagboom PE, et al.. Growth hormone secretion is diminished and tightly controlled in humans enriched for familial longevity. Aging Cell (2016) 15:1126–31. 10.1111/acel.12519 [PMC free article] [PubMed] [CrossRef] [Google Scholar]
- Sebastiani P, Sun FX, Andersen SL, Lee JH, Wojczynski MK, Sanders JL, et al.. Families enriched for exceptional longevity also have increased health-span: findings from the long life family study. Front Public Health (2013) 1:38. 10.3389/fpubh.2013.00038 [PMC free article] [PubMed] [CrossRef] [Google Scholar]
- Sebastiani P, Thyagarajan B, Sun F, Honig LS, Schupf N, Cosentino S, et al.. Age and sex distributions of age-related biomarker values in healthy older adults from the long life family study. J Am Geriatr Soc. (2016) 64:e189–94. 10.1111/jgs.14522 [PMC free article] [PubMed] [CrossRef] [Google Scholar]
- Teumer A, Qi Q, Nethander M, Aschard H, Bandinelli S, Beekman M, et al.. Genomewide meta-analysis identifies loci associated with IGF-I and IGFBP-3 levels with impact on age-related traits. Aging Cell (2016) 15:811–24. 10.1111/acel.12490 [PMC free article] [PubMed] [CrossRef] [Google Scholar]
- Deelen J, Uh HW, Monajemi R, van Heemst D, Thijssen PE, Böhringer S, et al .. Gene set analysis of GWAS data for human longevity highlights the relevance of the insulin / IGF-1 signaling and telomere maintenance pathways. Age (2013) 35: 235–49. 10.1007 / s11357-011-9340-3 [PMC free article] [PubMed] [CrossRef] [Google Scholar]
- van Heemst D, Beekman M, Mooijaart SP, Heijmans BT, Brandt BW, Zwaan BJ, et al .. Reduced insulin / IGF-1 signalling and human longevity. Aging Cell (2005) 4: 79–85. 10.1111 / j.1474-9728.2005.00148.x [PubMed] [CrossRef] [Google Scholar]
- Ben-Avraham D, Govindaraju DR, Budagov T, Fradin D, Durda P, Liu B, et al .. The GH receptor exon 3 deletion is a marker of male-specific exceptional longevity associated with increased GH sensitivity and taller stature. Sci Adv. (2017) 3: e1602025. 10.1126 / sciadv.1602025 [PMC free article] [PubMed] [CrossRef] [Google Scholar]
- McKiernan SH, Colman RJ, Lopez M, Beasley TM, Aiken JM, Anderson RM, et al .. Caloric restriction delays aging-induced cellular phenotypes in rhesus monkey skeletal muscle. Exp Gerontol. (2011) 46: 23–9. 10.1016 / j.exger.2010.09.011 [PMC free article] [PubMed] [CrossRef] [Google Scholar]
- Colman RJ, Anderson RM, Johnson SC, Kastman EK, Kosmatka KJ, Beasley TM, et al. Calorie restriction delays disease onset and mortality in rhesus monkeys. Science (2009) 325: 201-4. 10.1126 / science.1173635 [PMC free article] [PubMed] [CrossRef] [Google Scholar]
- Willcox DC, Willcox BJ, Todoriki H, Curb JD, Suzuki M. Caloric restriction and human longevity: what can we learn from the Okinawans? Biogerontology (2006) 7: 173–7. 10.1007 / s10522-006-9008-z [PubMed] [CrossRef] [Google Scholar]
- Mattison JA, Roth GS, Beasley TM, Tilmont EM, Handy AM, Herbert RL, et al .. Impact of caloric restriction on health and survival in rhesus monkeys from the NIA study. Nature (2012) 489: 318-21. 10.1038 / nature11432 [PMC free article] [PubMed] [CrossRef] [Google Scholar]
- Dunn SE, Kari FW, French J, Leininger JR, Travlos G, Wilson R, et al .. Dietary restriction reduces insulin-like growth factor I levels, which modulates apoptosis, cell proliferation, and tumor progression in p53-deficient mice. Cancer Res. (1997) 57: 4667–72. [PubMed] [Google Scholar]
- Berrigan D, Perkins SN, Haines DC, Hursting SD. Adult-onset calorie restriction and fasting delay spontaneous tumorigenesis in p53-deficient mice. Carcinogenesis (2002) 23: 817-22. 10.1093 / carcin / 23.5.817 [PubMed] [CrossRef] [Google Scholar]
- Redman LM, Veldhuis JD, Rood J, Smith SR, Williamson D, Ravussin E, et al .. The effect of caloric restriction interventions on growth hormone secretion in nonobese men and women. Aging Cell (2010) 9: 32–9. 10.1111 / j.1474-9726.2009.00530.x [PMC free article] [PubMed] [CrossRef] [Google Scholar]
- Fontana L, Villareal DT, Das SK, Smith SR, Meydani SN, Pittas AG, et al.. Effects of 2-year calorie restriction on circulating levels of IGF-1, IGF-binding proteins and cortisol in nonobese men and women: a randomized clinical trial. Aging Cell (2016) 15:22–7. 10.1111/acel.12400 [PMC free article] [PubMed] [CrossRef] [Google Scholar]
- Lettieri-Barbato D, Giovannetti E, Aquilano K. Effects of dietary restriction on adipose mass and biomarkers of healthy aging in human. Aging (2016) 8:3341–55. 10.18632/aging.101122 [PMC free article] [PubMed] [CrossRef] [Google Scholar]
- Mercken EM, Crosby SD, Lamming DW, JeBailey L, Krzysik-Walker S, Villareal DT, et al .. Calorie restriction in humans inhibits the PI3K / AKT pathway and induces a younger transcription profile. Aging Cell (2013) 12: 645-51. 10.1111 / acel.12088 [PMC free article] [PubMed] [CrossRef] [Google Scholar]
- Arai Y, Kojima T, Takayama M, Hirose N. The metabolic syndrome, IGF-1, and insulin action. Mol Cell Endocrinol. (2009) 299: 124–8. 10.1016 / j.mce.2008.07.002 [PubMed] [CrossRef] [Google Scholar]
- Redman LM, Ravussin E. Endocrine alterations in response to calorie restriction in humans. Mol Cell Endocrinol. (2009) 299: 129–36. 10.1016 / j.mce.2008.10.014 [PMC free article] [PubMed] [CrossRef] [Google Scholar]
- Franceschi C, Garagnani P, Vitale G, Capri M, Salvioli S. Inflammaging and 'Garb-aging'. Trends Endocrinol Metab. (2017) 28: 199–212. 10.1016 / j.tem.2016.09.09.005 [PubMed] [CrossRef] [Google Scholar]
- Qian M, Liu B. Pharmaceutical Intervention of Aging. Adv Exp Med Biol. (2018) 1086: 235–54. 10.1007 / 978-981-13-1117-8_15 [PubMed] [CrossRef] [Google Scholar]
- Baker DJ, Wijshake T, Tchkonia T, LeBrasseur NK, Childs BG, van de Sluis B, et al .. Clearance of p16Ink4a-positive senescent cells delays age-associated disorders. Nature (2011) 479: 232-6. 10.1038 / nature10600 [PMC free article] [PubMed] [CrossRef] [Google Scholar]
- Derous D, Mitchell SE, Wang L, Green CL, Wang Y, Chen L, et al .. The effects of graded levels of calorie restriction: XI. Evaluation of the main hypotheses underpinning the life extension effects of CR using the hepatic transcriptome. Aging (2017) 9: 1770–824. 10.18632 / aging.101269 [PMC free article] [PubMed] [CrossRef] [Google Scholar]
- Madeo F, Tavernarakis N, Kroemer G. Can autophagy promote longevity? Nat Cell Biol. (2010) 12: 842-6. 10.1038 / ncb0910-842 [PubMed] [CrossRef] [Google Scholar]
- Cevenini E, Monti D, Franceschi C. Inflamm-aging. Curr Opin Clin Nutr Metab Care. (2013) 16: 14–20. 10.1097 / MCO.0b013e32835ada13 [PubMed] [CrossRef] [Google Scholar]
- Meydani SN, Das SK, Pieper CF, Lewis MR, Klein S, Dixit VD, et al.. Long-term moderate calorie restriction inhibits inflammation without impairing cell-mediated immunity: a randomized controlled trial in non-obese humans. Aging (2016) 8:1416–31. 10.18632/aging.100994 [PMC free article] [PubMed] [CrossRef] [Google Scholar]
- Ott B, Skurk T, Hastreiter L, Lagkouvardos I, Fischer S, Büttner J, et al.. Effect of caloric restriction on gut permeability, inflammation markers, and fecal microbiota in obese women. Sci Rep. (2017) 7:11955. 10.1038/s41598-017-12109-9 [PMC free article] [PubMed] [CrossRef] [Google Scholar]
- Unamuno X, Gómez-Ambrosi J, Rodríguez A, Becerril S, Frühbeck G, Catalán V. Adipokine dysregulation and adipose tissue inflammation in human obesity. Eur J Clin Invest. (2018) 48:e12997. 10.1111/eci.12997 [PubMed] [CrossRef] [Google Scholar]
- Liu M, Liu F. Regulation of adiponectin multimerization, signaling and function. Best Pract Res Clin Endocrinol Metab. (2014) 28: 25–31. 10.1016 / j.beem.2013.06.003 [PMC free article] [PubMed] [CrossRef] [Google Scholar]
- Balaskó M, Soós S, Székely M, Pétervári E. Leptin and aging: Review and questions with particular emphasis on its role in the central regulation of energy balance. J Chem Neuroanat. (2014) 61–62: 248–55. 10.1016 / j.jchemneu.2014.08.00.006 [PubMed] [CrossRef] [Google Scholar]
- Arai Y, Nakazawa S, Kojima T, Takayama M, Abihara Y, Shimizu K, et al. High adiponectin concentration and its role for longevity in female centenarians. Geriatr Gerontol Int. (2006) 6: 32–9. 10.1111 / j.1447-0594.2006.00304.x [CrossRef] [Google Scholar]
- Bik W, Baranowska-Bik A, Wolinska-Witort E, Kalisz M, Broczek K, Mossakowska M, et al .. Assessment of adiponectin and its isoforms in Polish centenarians. Exp Gerontol. (2013) 48: 401-7. 10.1016 / j.exger.2013.01.015 [PubMed] [CrossRef] [Google Scholar]
- Meazza C, Vitale G, Pagani S, Castaldi D, Ogliari G, Mari D, et al .. Common adipokine features of neonates and centenarians. J Pediatr Endocrinol Metab. (2011) 24: 953–7. 10.1515 / JPEM.2011.373 [PubMed] [CrossRef] [Google Scholar]
- Baranowska B, Bik W, Baranowska-Bik A, Wolinska-Witort E, Szybinska A, Martynska L, et al .. Neuroendocrine control of metabolic homeostasis in Polish centenarians. J Physiol Pharmacol. (2006) 57 (Suppl. 6): 55–61. [PubMed] [Google Scholar]
- Miura Y, Hashii N, Tsumoto H, Takakura D, Ohta Y, Abe Y, et al .. Change in N-glycosylation of plasma proteins in Japanese semisupercentenarians. PLoS ONE (2015) 10: e0142645. 10.1371 / journal.pone.0142645 [PMC free article] [PubMed] [CrossRef] [Google Scholar]
- Atzmon G, Pollin TI, Crandall J, Tanner K, Schechter CB, Scherer PE, et al .. Adiponectin levels and genotype: a potential regulator of life span in humans. J Gerontol A Biol Sci Med Sci. (2008) 63: 447–53. 10.1093 / gerona / 63.5.447 [PMC free article] [PubMed] [CrossRef] [Google Scholar]
- Mariotti S, Barbesino G, Caturegli P, Bartalena L, Sansoni P, Fagnoni F, et al .. Complex alteration of thyroid function in healthy centenarians. J Clin. Endocrinol Metab. (1993) 77: 1130-4. 10.1210 / jcem.77.5.8077303 [PubMed] [CrossRef] [Google Scholar]
- Maugeri D, Russo MS, Di Stefano F, Receputo G, Rosso D, Rapisarda R, et al .. Thyroid function in healthy centenarians. Arch Gerontol Geriatr. (1997) 25: 211–7. 10.1016 / S0167-4943 (97) 00012-5 [PubMed] [CrossRef] [Google Scholar]
- Ostan R, Monti D, Mari D, Arosio B, Gentilini D, Ferri E, et al .. Heterogeneity of thyroid function and impact of peripheral thyroxine deiodination in centenarians and semi-supercentenarians: association with functional status and mortality. J Gerontol A Biol Sci Med Sci. (2018). 10.1093 / gerona / gly194. [Epub ahead of print]. [PubMed] [CrossRef] [Google Scholar]
- He Y, Chen X, Yan D, Xiao F, Liu Y, Lin R, et al .. Thyroid function decreases with age and may contribute to longevity in chinese centenarians' families. JAGS (2015) 63: 1474-6. 10.1111 / jgs.13553 [PubMed] [CrossRef] [Google Scholar]
- Corsonello A, Montesanto A, Berardelli M, De Rango F, Dato S, Mari V, et al .. A cross-section analysis of FT3 age-related changes in a group of old and oldest-old subjects, including centenarians' relatives, shows that a down-regulated thyroid function has a familial component and is related to longevity. Age Ageing (2010) 39: 723–7. 10.1093 / ageing / afq116 [PMC free article] [PubMed] [CrossRef] [Google Scholar]
- Pereira da Silva A, Matos A, Valente A, Gil Â, Alonso I, Ribeiro R, et al .. Body composition assessment and nutritional status evaluation in men and women portuguese centenarians. J Nutr Health Aging (2016) 20: 256–66. 10.1007 / s12603-015-0566-0 [PubMed] [CrossRef] [Google Scholar]
- Franceschi C, Ostan R, Santoro A. Nutrition and inflammation: are centenarians similar to individuals on calorie-restricted diets? Annu Rev Nutr. (2018) 38: 329–56. 10.1146 / annurev-nutr-082117-051637 [PubMed] [CrossRef] [Google Scholar]
- Gentilini D, Mari D, Castaldi D, Remondini D, Ogliari G, Ostan R, et al .. Role of epigenetics in human aging and longevity: genome-wide DNA methylation profile in centenarians and centenarians' offspring. Age (2013) 35: 1961–73. 10.1007 / s11357-012-9463-1 [PMC free article] [PubMed] [CrossRef] [Google Scholar]
- Sgarbi G, Matarrese P, Pinti M, Lanzarini C, Ascione B, Gibellini L, et al .. Mitochondria hyperfusion and elevated autophagic activity are key mechanisms for cellular bioenergetic preservation in centenarians. Aging (2014) 6: 296-310. 10.18632 / aging.100654 [PMC free article] [PubMed] [CrossRef] [Google Scholar]
- Emanuele E, Minoretti P, Sanchis-Gomar F, Pareja-Galeano H, Yilmaz Y, Garatachea N, et al .. Can enhanced autophagy be associated with human longevity? Serum levels of the autophagy biomarker beclin-1 are increased in healthy centenarians. Rejuvenation Res. (2014) 17: 518-24. 10.1089 / rej.2014.1607 [PubMed] [CrossRef] [Google Scholar]
- Raz Y, Guerrero-Ros I, Maier A, Slagboom PE, Atzmon G, Barzilai N, et al. Activation-induced autophagy is preserved in CD4 + T-cells in familial longevity. J Gerontol A Biol Sci Med Sci. (2017) 72: 1201-6. 10.1093 / gerona / glx020 [PMC free article] [PubMed] [CrossRef] [Google Scholar]
- Fontana L, Villareal DT, Weiss EP, Racette SB, Steger-May K, et al. Calorie restriction or exercise: effects on coronary heart disease risk factors. A randomized, controlled trial. Am J Physiol Endocrinol Metab. (2007) 293: E197–202. 10.1152 / ajpendo.00102.2007 [PubMed] [CrossRef] [Google Scholar]
- Ravussin E, Redman LM, Rochon J, Das SK, Fontana L, et al .. A 2-year randomized controlled trial of human caloric restriction: feasibility and effects on predictors of health span and longevity. J Gerontol A Biol Sci Med Sci. (2015) 70: 1097-104. 10.1093 / gerona / glv057 [PMC free article] [PubMed] [CrossRef] [Google Scholar]
- Bruunsgaard H, Andersen-Ranberg K, Jeune B, Pedersen AN, Skinhoj P, Pedersen BK. A high plasma concentration of TNF-α is associated with dementia in centenarians. J Gerontol A Biol Sci Med Sci. (1999) 54: M357–64. 10.1093 / gerona / 54.7.M357 [PubMed] [CrossRef] [Google Scholar]
- Gangemi S, Basile G, Merendino RA, Minciullo PL, Novick D, et al .. Increased circulating interleukin-18 levels in centenarians with no signs of vascular disease: another paradox of longevity? Exp Gerontol. (2003) 38: 669–72. 10.1016 / S0531-5565 (03) 00061-5 [PubMed] [CrossRef] [Google Scholar]
- Gerli R, Monti D, Bistoni O, Mazzone AM, Peri G, et al .. Chemokines, sTNF-Rs and sCD30 serum levels in healthy aged people and centenarians. Mech Ageing Dev. (2000) 121: 37–46. 10.1016 / S0047-6374 (00) 00195-0 [PubMed] [CrossRef] [Google Scholar]
- Franceschi C, Capri M, Monti D, Giunta S, Olivieri F, Sevini F, et al.. Inflammaging and anti-inflammaging: a systemic perspective on aging and longevity emerged from studies in humans. Mech Ageing Dev. (2007) 128:92–105. 10.1016/j.mad.2006.11.016 [PubMed] [CrossRef] [Google Scholar]
- Salvioli S, Monti D, Lanzarini C, Conte M, Pirazzini C, Bacalini MG, et al.. Immune system, cell senescence, aging and longevity–inflamm-aging reappraised. Curr Pharm Des. (2013) 19:1675–9. 10.2174/1381612811319090015
Источник
Giovanni Vitale, Giuseppe Pellegrino, Maria Vollery, and Leo J. Hofland. ROLE of IGF-1 System in the Modulation of Longevity: Controversies and New Insights From a Centenarians' Perspective Front Endocrinol (Lausanne). 2019; 10: 27.