Einstein Telescope: a new generation gravitational wave detector
Longer, more powerful, more precisely - Europe is going to build a new generation of gravitational-wave detector called Einstein Telescope .

Einstein Telescope concept art, credit: www.gwoptics.org
The AdvancedLIGO detector just started working a couple of years ago, and has not even reached its planned sensitivity. However, it is obvious to scientists that the sensitivity of LIGO will not be enough for real gravitational-wave astronomy.
I will talk about what limits LIGO, and how an underground cryogenic detector 2.5 times longer than LIGO can bypass these restrictions.
First, I briefly recall how LIGO detects gravitational waves, and define some concepts.
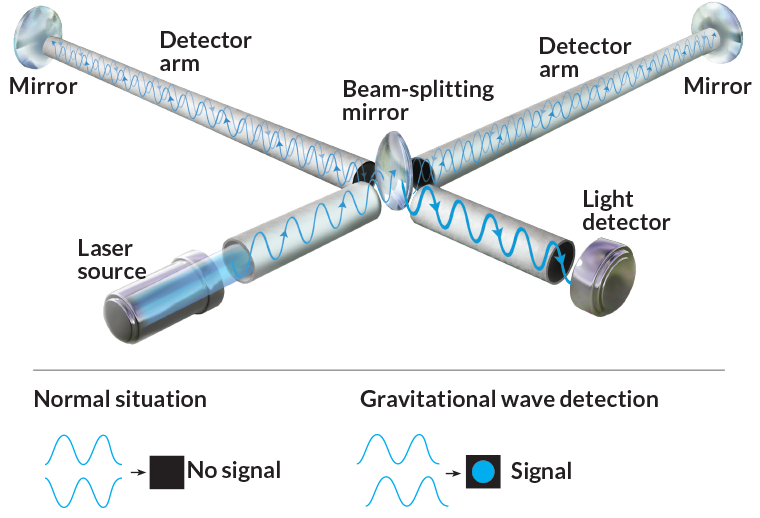
The LIGO detector is a Michelson interferometer. Gravitational waves stretch one shoulder and squeeze the other, the relative phase of the light on the beam splitter changes, and an interference pattern appears at the output. Image credit: induced.info
Gravitational waves (GW) are small perturbations of the space-time metric. They arise when asymmetrical movement of massive bodies, for example, when two black holes merge. These perturbations lead to a change in the definition of the distance between the object (“stretch” and “compress” the distance). The gravitational-wave detector is designed so that it allows you to measure this change in distance with the help of lasers. In the simplest version, the detector is a Michelson interferometer, where the detector arms are balanced so that due to constructive interference all light is reflected towards the source, and the second output of the beam divider due to destructive interference remains dark.
When the GWs reach the detector, they stretch one shoulder and compress the other, which changes the interference pattern at the output of the interferometer and allows you to register the signal.
In the last article I explained that the GW detector is not a ruler, but a clock, i.e. measures the relative delay of light in two shoulders caused by a gravitational wave. I also showed that the relative change in the phase of light:
This equation explains why the detectors are made so long: it allows you to increase the sensitivity.
To further increase the sensitivity of scientists invented the use of optical resonators. They allow light to travel in the shoulder several times.
, effectively increasing the length of the shoulder in
time.
Also, the signal at the output of the detector is proportional to the light power inside the detector, so that the resonators solve two problems at once, because they increase the light power.
Gravitational waves have a polarization: they can be either “+” (relative to the detector - they stretch one shoulder and squeeze the other), or “x” (stretch / squeeze both shoulders at the same time).

The displacement of the test mass (balls) under the action of GW of different polarizations during the same period. Credit: [Tiec, Novak, 2017]
The detector is sensitive only to “+” polarization. Therefore, it is important to have several detectors with slightly different shoulder orientations in order to measure waves of any polarization: if one detector is oriented towards “+” and the other towards x, then if one detector sees the wave and the other does not, we are sure that this polarization was exactly “+”. And if both of them saw a wave of different amplitude, then we can calculate what the initial polarization was.
Sensitivity to polarization sets a different radiation pattern for two polarizations (that is, which points in the sky are best seen by the detector).

The directivity pattern of the detector to x and + polarizations, as well as averaged over two polarizations. Credit: arXiv: 1501.03765
LIGO has an incredible sensitivity: allows you to measure the relative change in the length of the shoulders with an accuracy of 10 -18 m.
To measure signals with such accuracy, it is necessary to get rid of all sorts of noise in different parts of the instrument.
The sensitivity of the detector is usually shown as the noise level in the detector at different frequencies in the form of spectral density. The spectral density reflects the contribution of different noise to the signal at the detector output (i.e. some noise may be significant at the place of origin, but make a small contribution to the noise at the output). Typically, the spectral density is normalized to the amplitude of gravitational waves (which is called strain,
)

The main contributions to the sensitivity of LIGO at different frequencies, normalized to the amplitude of the GW strain,
Consider some of the most important contributions to noise:
1. Seismic noise (limits frequencies <1 Hz): any seismic activity can displace mirrors. To isolate from this noise, the mirrors are suspended on a multi-stage suspension, which in turn is fixed on a multi-level massive stand. The lower the resonant frequency of the suspension, the more suppressed noise at low frequencies. In principle, there are no restrictions on the quality of noise suppression.
2. Newton's gravitational noise(limits frequencies ~ 1 Hz): even if the mirrors are completely isolated from direct seismic impact, the displacement of the ground / floor surface can affect the mirrors gravitationally. Acoustic waves propagating over the surface of the earth, for example, from wind or waves, slightly change the distance from the mirror to the ground, and hence the force of gravity, which can displace the mirror. Isolate completely from this is impossible, this is a fundamental limitation.
3. Thermal noise of suspensions (limits frequencies ~ 1-10 Hz): thermal movement of molecules in the suspensions of mirrors leads to the excitation of oscillations in the suspension, which displaces the mirrors. It is difficult to suppress, it all depends on the quality of materials.
4. Thermal noise of mirrors(limits sensitivity from below): thermal movement of molecules in the coatings of mirrors, and in the very “body” of mirrors (substrate). It looks to the beam of light as the displacement of the mirror itself. Limited by materials, the most important technical noise.
5. Quantum shot noise of the laser (frequency> 50 Hz): the light has a quantum nature, separate photons fly with a different random delay. This delay is visible as a phase measurement at the output of the interferometer, and limits all frequencies. The greater the power of light inside the detector, the less noise. The fundamental limit, but can be suppressed with the help of squeezed light.
6. Quantum noise radiation pressure(frequencies 10-50Hz): the same shot noise causes power fluctuations inside the interferometer and causes the random force of radiation pressure on the mirrors. As fundamental as shot noise. Unlike shot noise, it increases with increasing light power.

An explanation about quantum noise. Single photons produce a random radiation pressure force (left). On the other hand, the random distribution of photons over time leads to amplitude fluctuations on the photodetector (right). Both noise depend on the wavelength, light power and shoulder length. The noise of radiation pressure is smaller, the greater the mass of the mirrors. Credit: [1].

Sensitivity versus light power
: shot noise (blue) decreases, while radiation pressure noise (green) increases proportionally.
7. Residual gas in the vacuum system (all frequencies, but not limited now): the ultra-high vacuum in the system is not always perfect, and the residual gas molecules can scatter light. It may be arbitrarily small (depending on the quality of the pumps).
8. Classic laser noises (do not limit): the power and frequency of the laser can also fluctuate for classical reasons (thermal noise, vibrations). The laser system includes ultra-stable lasers and multi-level systems for controlling the frequency and power of the laser.
All these noises can be divided into two groups: force - fluctuations lead to physical displacement of mirrors (noise 1-3 and 6), and coordinate - fluctuations lead to a change in the phase of light, but do not displace mirrors (noise 4.5 and 7).
Power noise
cause offset
test masses according to Newton's law
or in the frequency range:
. That is, these noises can be reduced by increasing the mass of the mirrors.
The LIGO design fundamentally cannot solve the problem of Newtonian noise 2, and without a complete restructuring of the optical systems, the problem of thermal noise of mirrors 4.
In the details about the noise can be read in the wonderful article about LIGO in Habré .

The KAGRA Underground Detector will join the observations next year.
So, the new detector will be located underground. This will reduce seismic noise 1, and, most importantly, Newtonian noise 2: the main contribution to it is caused by surface waves, which are almost not underground.
Depending on where the detector will be built (now there are two main options - in the Netherlands or in Sardinia, and possibly in Hungary).

Seismic comparison in different possible locations with AdvancedVirgo detector in Italy.
Of course, the most obvious technical steps to suppress seismic will be made: a new suspension system for passive isolation and heavier mirrors of 200 kg each to suppress all power noise.

One of the corner stations of the Einstein telescope with a variety of vacuum chambers. Credit: gwoptics.org
The problem of thermal noise of mirrors is more complicated. An obvious solution would be to cool the mirrors, thereby reducing the Brownian noise.
However, cooling will lead to a change in the optical properties of the mirrors, and increase the absorption. In addition, with cold mirrors it is impossible to use large light powers: the absorption in the mirrors will heat them up and reduce cooling to nothing. That is, you need to cool the detector and reduce the power of the light? It also will not work this way - shot noise will increase (4), and will spoil the sensitivity at low frequencies.
Scientists have come to another solution: use two interferometers in one place.
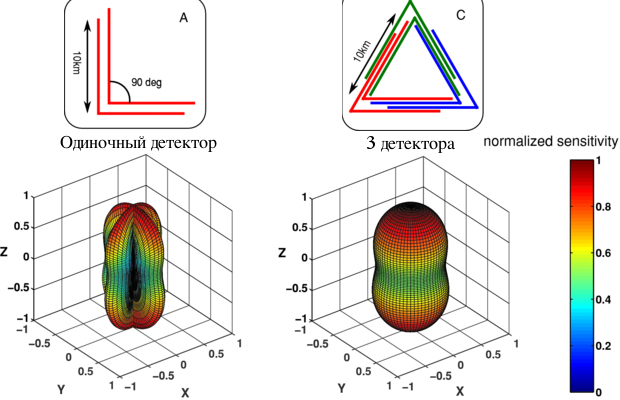
"Xylophone" detector configuration with two interferometers nested into each other. Credit: A. Freise et al, CQG 26 (2009) 085012
One will be optimized for low frequencies, work with mirrors cooled to 20K, and use low light power. Shot noise will increase, but the detector will not be used at frequencies where shot noise is significant. The second detector will operate at room temperature at high power: this will suppress shot noise at high frequencies, but will spoil the sensitivity at low frequencies with increased radiation pressure noise. But this detector will not be used at low frequencies. As a result, the combined sensitivity will be optimal at all frequencies.

ET-D-LF low-frequency detector with chilled mirrors and low power (and low radiation pressure noise), and high power ET-D-HF high power (and low shot noise). Credit: [1]
Another problem with the new generation of detectors: at the time of construction, it will be the only one with such sensitivity. First, it will not be possible to distinguish a random burst from a signal if it is not possible to check the coincidence between the detectors. Secondly, it will not be possible to measure different polarizations of gravitational waves. Scientists propose to build not one detector, but three with different orientations (in the form of a triangle, as in the picture).
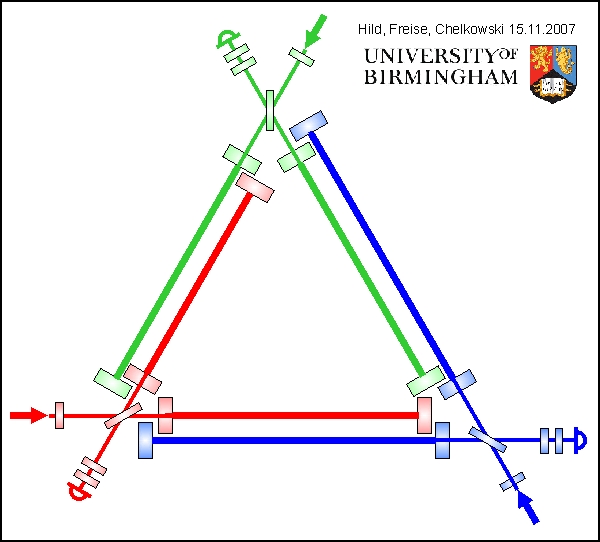

The concept of a triangular detector configuration (left); tunnels with different shoulders (right).
This will improve the detector radiation pattern and record much more events:

Comparison of the radiation pattern of one detector (left) and three detectors in a triangular configuration (right).
Let me remind you that each of them will consist of two: one for low frequencies and the other for high frequencies. As a result, six detectors will be located a triangle.

All these tricks will increase the sensitivity of the detectors by at least an order of magnitude.
Such sensitivity will allow to increase the observation range practically to the border of the visible Universe, to see the BH fusions of the first generation of stars and to observe the fusions of black holes and neutron stars constantly.
Increasing the sensitivity at low frequencies allows observing the earlier stages of merging objects, and getting more information about their parameters.
High frequencies will allow to observe the evolution of a black hole or a neutron star, formed as a result of a merger. This mode is most interesting for checking GTR and possible alternatives. For example, gravitational-wave echo can be observed at high frequencies.

Comparing the sensitivity of ET and LIGO-Virgo
But the most important thing is that it will be not just a detector, but a whole infrastructure that will allow the detector to increase its sensitivity for many decades.
I have not yet discussed such an important part of ET as a system for suppressing quantum noise using frequency dependent squeezed light. You can read more about squeezed light in a great article on Habré . I plan to tell you more about the quantum noise in the detector in the next article.
In addition, ET will use the so-called optical rigidity - signal amplification due to the nonlinear interaction between the mechanical oscillator and the light inside the resonators. More about quantum optomechanics - the science of the interaction between mechanical systems and light - soon on Habré;)
Of course, I touched on only the most basic features of ET, there are a lot of details - welcome to the comments.
In addition, I did not mention that in the USA it is planned to build an even longer 40km ground-based telescope Cosmic Explorer , but its design is still less elaborated than the ET, so I will not tell any interesting details.

Currently ET has not yet received the approval of the European Commission. Individual countries invest in preliminary research. Collaboration is gradually being formed. You can read the official website and even join the collaboration by signing the Letter of Intent .
Under the plan, in the next year or two, Europe will consider the application for creation and approve the location. The launch of ET in this case will occur in the early 2030s.

One of the options is a triangle on the border of Germany, Belgium and the Netherlands, located so that in each country there will be one corner station. It will be a symbol of a united Europe.
In the meantime, LIGO announced the results of data processing from the previous O2 observation cycle: there were four more new black hole fusions. Thus, for all the time, LIGO has already seen 10 black hole fusions and one neutron star fusion. Tomorrow all the data will be officially presented, and I will add some details to the article.
UPD: So, the new catalog of gravitational waves was published on arXiv , along with an updated analysis of the data for all events. There are no sensational discoveries, but we have already seen 10 mergers of black holes, and this in itself is remarkable.

All black holes (solar masses) and neutron stars known to us, including observations of LIGO-Virgo. You can see online.Credit: LIGO-Virgo / Frank Elavsky / Northwestern
In the meantime, the detectors are being updated to increase their sensitivity, and in the spring of 2019 the detectors will be launched in the new O3 annual observation cycle. The sensitivity will be so great that it is planned to observe an average of one event per week. In the summer of 2019, according to the plan, a Japanese detector KARGA will join the two LIGO detectors and the Virgo detector.
This O3 cycle will be interesting for open science, since now all potential candidates for mergers will be announced in real time along with an assessment of their source, which will allow all interested to make observations in other ranges. Read more here .
The era of gravitational-wave astronomy is just beginning, there is a lot of interesting things ahead. Stay tuned!
I also invite you to read previous publications, where I tell you how important it is to observe neutron stars in GWs , what interesting physics allow us to study black hole fusions, and how LIGO can work at all if GW stretch light along with space.

Einstein Telescope concept art, credit: www.gwoptics.org
The AdvancedLIGO detector just started working a couple of years ago, and has not even reached its planned sensitivity. However, it is obvious to scientists that the sensitivity of LIGO will not be enough for real gravitational-wave astronomy.
I will talk about what limits LIGO, and how an underground cryogenic detector 2.5 times longer than LIGO can bypass these restrictions.
1. Introduction about the principles of operation of the GW detector
First, I briefly recall how LIGO detects gravitational waves, and define some concepts.
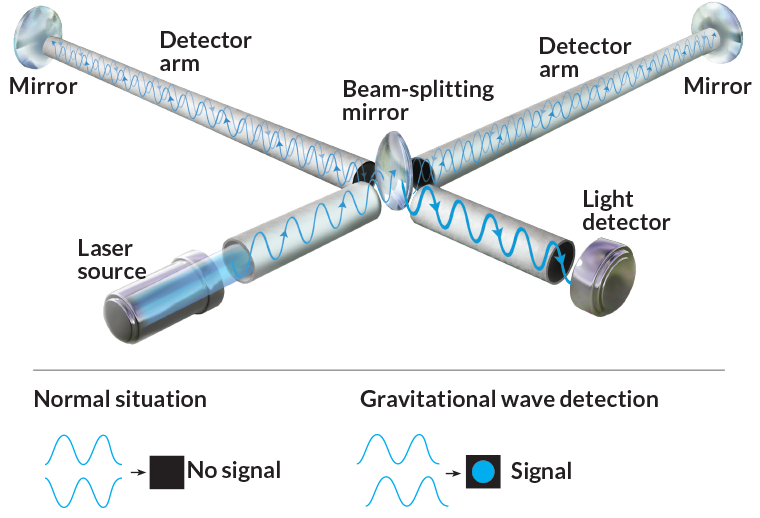
The LIGO detector is a Michelson interferometer. Gravitational waves stretch one shoulder and squeeze the other, the relative phase of the light on the beam splitter changes, and an interference pattern appears at the output. Image credit: induced.info
1.1 Principle of operation
Gravitational waves (GW) are small perturbations of the space-time metric. They arise when asymmetrical movement of massive bodies, for example, when two black holes merge. These perturbations lead to a change in the definition of the distance between the object (“stretch” and “compress” the distance). The gravitational-wave detector is designed so that it allows you to measure this change in distance with the help of lasers. In the simplest version, the detector is a Michelson interferometer, where the detector arms are balanced so that due to constructive interference all light is reflected towards the source, and the second output of the beam divider due to destructive interference remains dark.
When the GWs reach the detector, they stretch one shoulder and compress the other, which changes the interference pattern at the output of the interferometer and allows you to register the signal.
In the last article I explained that the GW detector is not a ruler, but a clock, i.e. measures the relative delay of light in two shoulders caused by a gravitational wave. I also showed that the relative change in the phase of light:
This equation explains why the detectors are made so long: it allows you to increase the sensitivity.
To further increase the sensitivity of scientists invented the use of optical resonators. They allow light to travel in the shoulder several times.
Also, the signal at the output of the detector is proportional to the light power inside the detector, so that the resonators solve two problems at once, because they increase the light power.
1.2 Polarization of gravitational waves
Gravitational waves have a polarization: they can be either “+” (relative to the detector - they stretch one shoulder and squeeze the other), or “x” (stretch / squeeze both shoulders at the same time).

The displacement of the test mass (balls) under the action of GW of different polarizations during the same period. Credit: [Tiec, Novak, 2017]
The detector is sensitive only to “+” polarization. Therefore, it is important to have several detectors with slightly different shoulder orientations in order to measure waves of any polarization: if one detector is oriented towards “+” and the other towards x, then if one detector sees the wave and the other does not, we are sure that this polarization was exactly “+”. And if both of them saw a wave of different amplitude, then we can calculate what the initial polarization was.
Sensitivity to polarization sets a different radiation pattern for two polarizations (that is, which points in the sky are best seen by the detector).

The directivity pattern of the detector to x and + polarizations, as well as averaged over two polarizations. Credit: arXiv: 1501.03765
2. Limitations of LIGO
LIGO has an incredible sensitivity: allows you to measure the relative change in the length of the shoulders with an accuracy of 10 -18 m.
To measure signals with such accuracy, it is necessary to get rid of all sorts of noise in different parts of the instrument.
The sensitivity of the detector is usually shown as the noise level in the detector at different frequencies in the form of spectral density. The spectral density reflects the contribution of different noise to the signal at the detector output (i.e. some noise may be significant at the place of origin, but make a small contribution to the noise at the output). Typically, the spectral density is normalized to the amplitude of gravitational waves (which is called strain,

The main contributions to the sensitivity of LIGO at different frequencies, normalized to the amplitude of the GW strain,
Consider some of the most important contributions to noise:
1. Seismic noise (limits frequencies <1 Hz): any seismic activity can displace mirrors. To isolate from this noise, the mirrors are suspended on a multi-stage suspension, which in turn is fixed on a multi-level massive stand. The lower the resonant frequency of the suspension, the more suppressed noise at low frequencies. In principle, there are no restrictions on the quality of noise suppression.
2. Newton's gravitational noise(limits frequencies ~ 1 Hz): even if the mirrors are completely isolated from direct seismic impact, the displacement of the ground / floor surface can affect the mirrors gravitationally. Acoustic waves propagating over the surface of the earth, for example, from wind or waves, slightly change the distance from the mirror to the ground, and hence the force of gravity, which can displace the mirror. Isolate completely from this is impossible, this is a fundamental limitation.
3. Thermal noise of suspensions (limits frequencies ~ 1-10 Hz): thermal movement of molecules in the suspensions of mirrors leads to the excitation of oscillations in the suspension, which displaces the mirrors. It is difficult to suppress, it all depends on the quality of materials.
4. Thermal noise of mirrors(limits sensitivity from below): thermal movement of molecules in the coatings of mirrors, and in the very “body” of mirrors (substrate). It looks to the beam of light as the displacement of the mirror itself. Limited by materials, the most important technical noise.
5. Quantum shot noise of the laser (frequency> 50 Hz): the light has a quantum nature, separate photons fly with a different random delay. This delay is visible as a phase measurement at the output of the interferometer, and limits all frequencies. The greater the power of light inside the detector, the less noise. The fundamental limit, but can be suppressed with the help of squeezed light.
6. Quantum noise radiation pressure(frequencies 10-50Hz): the same shot noise causes power fluctuations inside the interferometer and causes the random force of radiation pressure on the mirrors. As fundamental as shot noise. Unlike shot noise, it increases with increasing light power.

An explanation about quantum noise. Single photons produce a random radiation pressure force (left). On the other hand, the random distribution of photons over time leads to amplitude fluctuations on the photodetector (right). Both noise depend on the wavelength, light power and shoulder length. The noise of radiation pressure is smaller, the greater the mass of the mirrors. Credit: [1].

Sensitivity versus light power
7. Residual gas in the vacuum system (all frequencies, but not limited now): the ultra-high vacuum in the system is not always perfect, and the residual gas molecules can scatter light. It may be arbitrarily small (depending on the quality of the pumps).
8. Classic laser noises (do not limit): the power and frequency of the laser can also fluctuate for classical reasons (thermal noise, vibrations). The laser system includes ultra-stable lasers and multi-level systems for controlling the frequency and power of the laser.
All these noises can be divided into two groups: force - fluctuations lead to physical displacement of mirrors (noise 1-3 and 6), and coordinate - fluctuations lead to a change in the phase of light, but do not displace mirrors (noise 4.5 and 7).
Power noise
The LIGO design fundamentally cannot solve the problem of Newtonian noise 2, and without a complete restructuring of the optical systems, the problem of thermal noise of mirrors 4.
In the details about the noise can be read in the wonderful article about LIGO in Habré .
3. How will the new detector solve these problems?

The KAGRA Underground Detector will join the observations next year.
So, the new detector will be located underground. This will reduce seismic noise 1, and, most importantly, Newtonian noise 2: the main contribution to it is caused by surface waves, which are almost not underground.
Depending on where the detector will be built (now there are two main options - in the Netherlands or in Sardinia, and possibly in Hungary).

Seismic comparison in different possible locations with AdvancedVirgo detector in Italy.
Of course, the most obvious technical steps to suppress seismic will be made: a new suspension system for passive isolation and heavier mirrors of 200 kg each to suppress all power noise.

One of the corner stations of the Einstein telescope with a variety of vacuum chambers. Credit: gwoptics.org
The problem of thermal noise of mirrors is more complicated. An obvious solution would be to cool the mirrors, thereby reducing the Brownian noise.
However, cooling will lead to a change in the optical properties of the mirrors, and increase the absorption. In addition, with cold mirrors it is impossible to use large light powers: the absorption in the mirrors will heat them up and reduce cooling to nothing. That is, you need to cool the detector and reduce the power of the light? It also will not work this way - shot noise will increase (4), and will spoil the sensitivity at low frequencies.
Scientists have come to another solution: use two interferometers in one place.
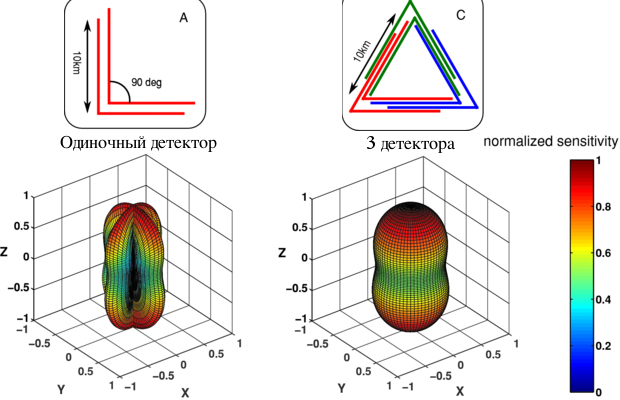
"Xylophone" detector configuration with two interferometers nested into each other. Credit: A. Freise et al, CQG 26 (2009) 085012
One will be optimized for low frequencies, work with mirrors cooled to 20K, and use low light power. Shot noise will increase, but the detector will not be used at frequencies where shot noise is significant. The second detector will operate at room temperature at high power: this will suppress shot noise at high frequencies, but will spoil the sensitivity at low frequencies with increased radiation pressure noise. But this detector will not be used at low frequencies. As a result, the combined sensitivity will be optimal at all frequencies.

ET-D-LF low-frequency detector with chilled mirrors and low power (and low radiation pressure noise), and high power ET-D-HF high power (and low shot noise). Credit: [1]
Another problem with the new generation of detectors: at the time of construction, it will be the only one with such sensitivity. First, it will not be possible to distinguish a random burst from a signal if it is not possible to check the coincidence between the detectors. Secondly, it will not be possible to measure different polarizations of gravitational waves. Scientists propose to build not one detector, but three with different orientations (in the form of a triangle, as in the picture).
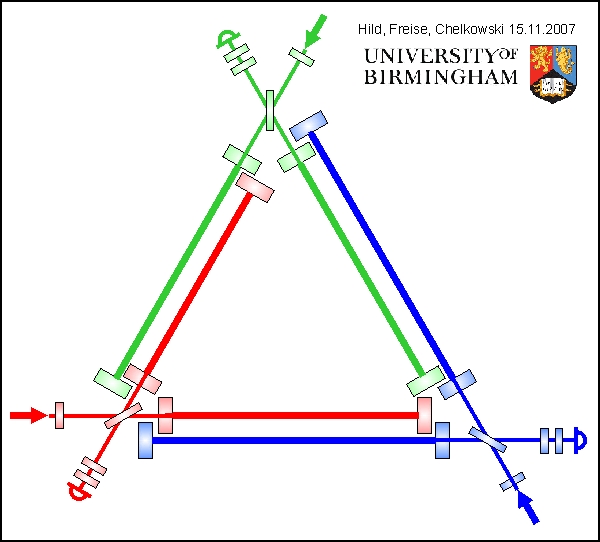

The concept of a triangular detector configuration (left); tunnels with different shoulders (right).
This will improve the detector radiation pattern and record much more events:

Comparison of the radiation pattern of one detector (left) and three detectors in a triangular configuration (right).
Let me remind you that each of them will consist of two: one for low frequencies and the other for high frequencies. As a result, six detectors will be located a triangle.

All these tricks will increase the sensitivity of the detectors by at least an order of magnitude.
Such sensitivity will allow to increase the observation range practically to the border of the visible Universe, to see the BH fusions of the first generation of stars and to observe the fusions of black holes and neutron stars constantly.
Increasing the sensitivity at low frequencies allows observing the earlier stages of merging objects, and getting more information about their parameters.
High frequencies will allow to observe the evolution of a black hole or a neutron star, formed as a result of a merger. This mode is most interesting for checking GTR and possible alternatives. For example, gravitational-wave echo can be observed at high frequencies.

Comparing the sensitivity of ET and LIGO-Virgo
But the most important thing is that it will be not just a detector, but a whole infrastructure that will allow the detector to increase its sensitivity for many decades.
4. Conclusion
What I did not mention
I have not yet discussed such an important part of ET as a system for suppressing quantum noise using frequency dependent squeezed light. You can read more about squeezed light in a great article on Habré . I plan to tell you more about the quantum noise in the detector in the next article.
In addition, ET will use the so-called optical rigidity - signal amplification due to the nonlinear interaction between the mechanical oscillator and the light inside the resonators. More about quantum optomechanics - the science of the interaction between mechanical systems and light - soon on Habré;)
Of course, I touched on only the most basic features of ET, there are a lot of details - welcome to the comments.
In addition, I did not mention that in the USA it is planned to build an even longer 40km ground-based telescope Cosmic Explorer , but its design is still less elaborated than the ET, so I will not tell any interesting details.
Einstein Telescope Status

Currently ET has not yet received the approval of the European Commission. Individual countries invest in preliminary research. Collaboration is gradually being formed. You can read the official website and even join the collaboration by signing the Letter of Intent .
Under the plan, in the next year or two, Europe will consider the application for creation and approve the location. The launch of ET in this case will occur in the early 2030s.

One of the options is a triangle on the border of Germany, Belgium and the Netherlands, located so that in each country there will be one corner station. It will be a symbol of a united Europe.
LIGO news
In the meantime, LIGO announced the results of data processing from the previous O2 observation cycle: there were four more new black hole fusions. Thus, for all the time, LIGO has already seen 10 black hole fusions and one neutron star fusion. Tomorrow all the data will be officially presented, and I will add some details to the article.
UPD: So, the new catalog of gravitational waves was published on arXiv , along with an updated analysis of the data for all events. There are no sensational discoveries, but we have already seen 10 mergers of black holes, and this in itself is remarkable.

All black holes (solar masses) and neutron stars known to us, including observations of LIGO-Virgo. You can see online.Credit: LIGO-Virgo / Frank Elavsky / Northwestern
In the meantime, the detectors are being updated to increase their sensitivity, and in the spring of 2019 the detectors will be launched in the new O3 annual observation cycle. The sensitivity will be so great that it is planned to observe an average of one event per week. In the summer of 2019, according to the plan, a Japanese detector KARGA will join the two LIGO detectors and the Virgo detector.
This O3 cycle will be interesting for open science, since now all potential candidates for mergers will be announced in real time along with an assessment of their source, which will allow all interested to make observations in other ranges. Read more here .
The era of gravitational-wave astronomy is just beginning, there is a lot of interesting things ahead. Stay tuned!
I also invite you to read previous publications, where I tell you how important it is to observe neutron stars in GWs , what interesting physics allow us to study black hole fusions, and how LIGO can work at all if GW stretch light along with space.
Literature
[1] S. Hild Beyond 2nd Generation GW detectors
[2] ET Design Study
[3] LIGO Open Science Data Center.
[2] ET Design Study
[3] LIGO Open Science Data Center.