Ask Ethan: If dark matter is everywhere, why haven't we found it in the solar system?
- Transfer

Halo of dark matter with lumpiness with various densities and a huge, scattered structure that simulations predict. For scale, the light part of the galaxy is shown. Since dark matter is everywhere, it must be in our solar system. So why haven't we seen it yet?
According to the vast amount of evidence, most of the universe consists of some mysterious mass of the type, which we have never measured directly. Protons, neutrons and electrons — and in general all matter consisting of particles that make up the Standard Model — of which the planets, stars, and galaxies that we find throughout the entire Universe consist of only 15% of its total mass. The rest consists of something completely different: cold dark matter. But if dark matter is everywhere and in large quantities, why have we not seen in the solar system? This is the question our reader asks:
All evidence of the presence of dark matter and dark energy belong to a distant cosmos. It is rather suspicious that we see no evidence of their existence here in our solar system. No one has ever reported any anomalies in the orbits of the planets. However, they are very accurately measured. If the universe is 95% dark, such effects could be measured locally.Is it so? It was one of the first thoughts that came to my mind when I first learned about dark matter (TM) 17 years ago. Let's understand and find out the truth.
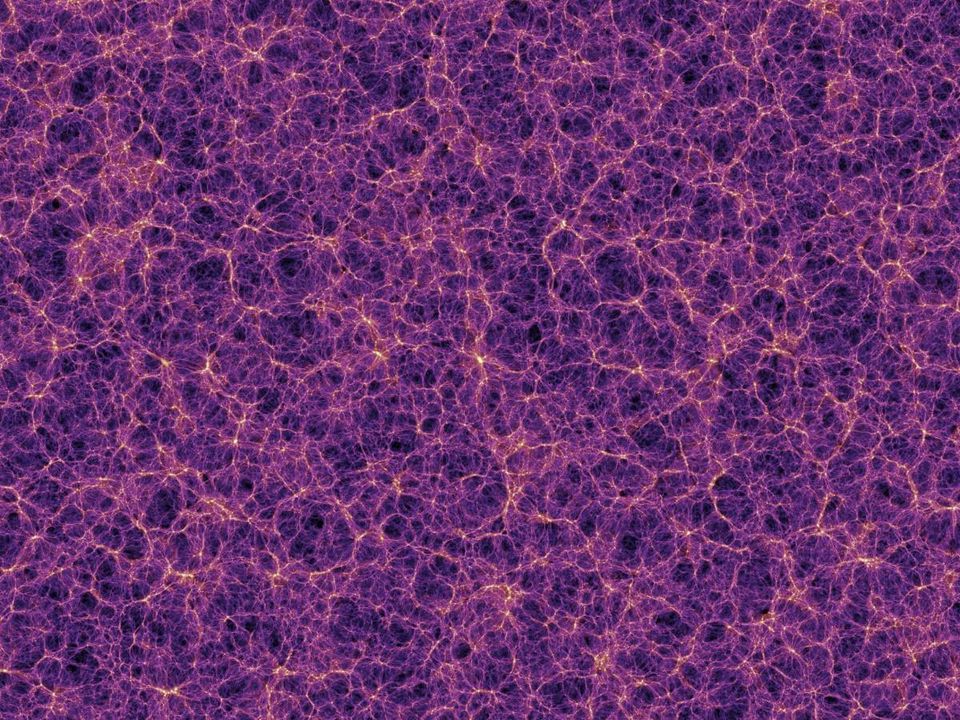
The cosmic web of dark matter and the large-scale structure it forms. Normal matter is present, but is only 1/6 of the total amount of matter. The remaining 5/6 is dark matter, and no amount of ordinary matter can cope with this.
The main idea of TM is that at some point in a very young Universe, before the appearance of galaxies, stars or even neutral atoms, there was an almost perfect and smooth TM sea, distributed throughout the space. Over time, gravity and other forces went through several interrelated stages:
- all matter, normal and dark, is attracted by gravity,
- sections with a density above the average grow, attracting both types of matter,
- radiation collides with normal matter and puts pressure on it,
- but this does not happen with TM, at least it does not occur in exactly the same way.
This creates a very specific pattern of areas of high and low density in the Universe; a pattern that manifests when we look at relic radiation (RI).
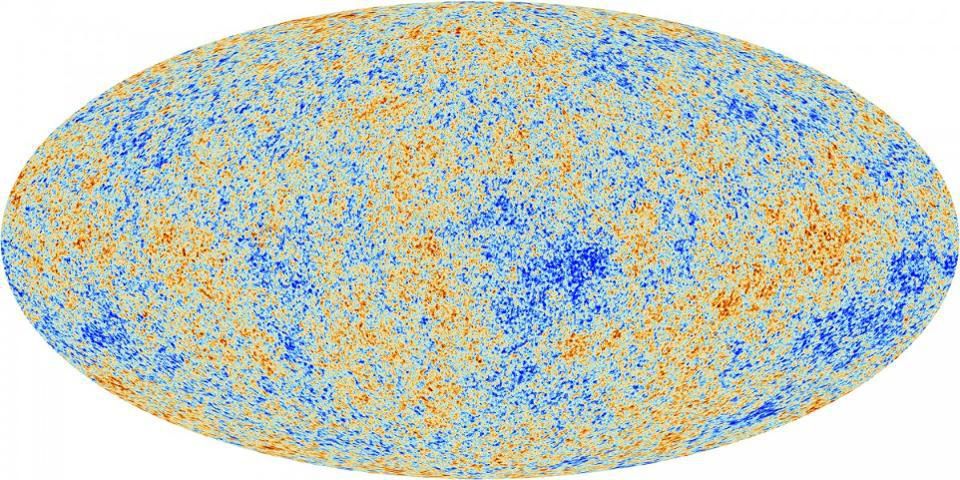
RI fluctuations are so small and have such a characteristic appearance that they convincingly indicate that the Universe at the very beginning had the same temperature everywhere, and also contained dark matter, ordinary matter and dark energy in certain proportions.
RI is the residual glow of the Big Bang: radiation that fell into our eyes, having traveled from the moment when stable neutral atoms first formed in the Universe. Today we see a photograph of the Universe as we move from an ionized plasma to an electrically neutral set of atoms, when the radiation pressure becomes negligible. Cold areas correspond to regions of increased density, since radiation has to spend extra energy (more than average) to get out of these gravity wells; hot areas - respectively, regions with low density.
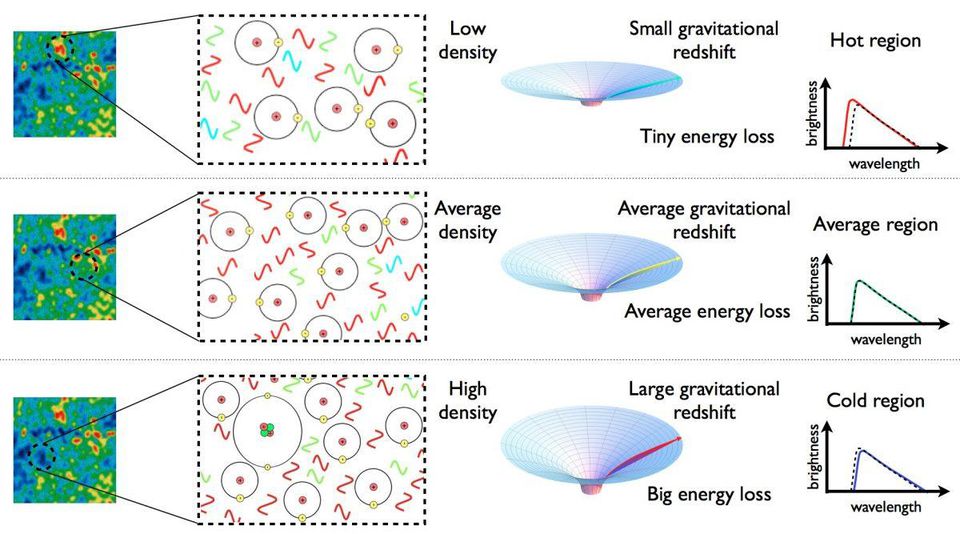
The high, medium, and low density areas that existed when the Universe was only 380,000 years old now correspond to cold, medium, and hot RI areas.
The picture of cold and hot areas on all scales that we can observe, and the correlation between them, tell us about the composition of the Universe: 68% of dark energy, 27% of TM, 5% of normal matter. Over time, these areas of increased density grew into stars, star clusters, galaxies and galactic clusters, and areas of low density gave up their matter to the surrounding areas of increased density. And although we can only see normal matter, due to the fact that it emits and interacts with light and other types of radiation, TM is the dominant force responsible for the gravitational growth of the structures of the Universe.

A careful study of the Universe demonstrates that it consists of matter, not antimatter, that TM and dark energy are necessary, and that we do not know the sources of all these mysteries. However, the RI fluctuations, formation and correlations between large-scale structures, and modern observations of gravitational lensing point to the same picture.
Since normal matter interacts with itself, gravitational collapse for normal and dark matter occurs in different ways. A lump of normal matter, gathered under the influence of gravity, begins to shrink. Compression first comes from the shortest dimension, but normal matter interacts and collides with other particles of normal matter - just like your hands, although they are composed of atoms that are almost empty space, will clap when you try to hold one hand through another. This interaction leads to the appearance of a rotating disk of matter - it is from it that everything flows, from disk (spiral) galaxies to solar systems, the planets in which move in orbits lying in the same plane. Dark matter, on the other hand, does not collide with itself, nor with normal matter, which is why it remains in the form of large and extremely rarefied halos. And although dark matter is more than usual, its density, for example, in our galaxy, is much less in those places where there are stars.
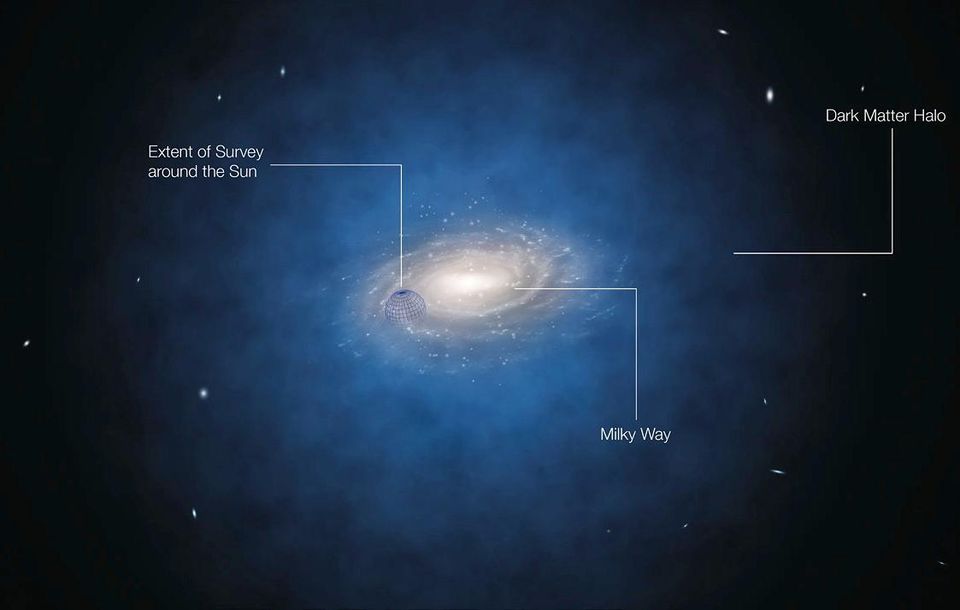
During the Earth’s revolution around the Sun, our movement through the TM in our galaxy changes, therefore its halo should demonstrate various interaction properties.
And now we come to the main issue. How does TM affect the solar system? Most of what you are likely to imagine will somehow be true: particles of TM must fly in space everywhere, including the entire space of the Milky Way. And this means that TM should be in the Solar System, in the Sun, should pass through our planet and our bodies. The big question is: compared with the masses of the Sun, planets, other objects of the Solar system, what will be the mass of TM of interest to us?

In the solar system in the first approximation of the orbits of the planets determines the sun. In the second approximation, all other masses (planets, moons, asteroids, etc.) play an important role. But to add more TM here, you need to greatly increase accuracy.
To answer, we need to first understand what determines the orbits of objects inside our solar system. With a large margin, the dominant mass in the solar system will be the sun. With a very precise approximation, it determines the orbits of the planets. But for Venus, the planet Mercury will be internal, and as a first approximation, the total mass of the Sun and Mercury determine the orbit of Venus. The orbit of Jupiter is determined by the sum of the mass of the Sun and all the inner planets, as well as the asteroid belt. For any object as a whole, its orbit is determined by the total mass enclosed in an imaginary sphere with the center in the sun and this object on the edge of the sphere.
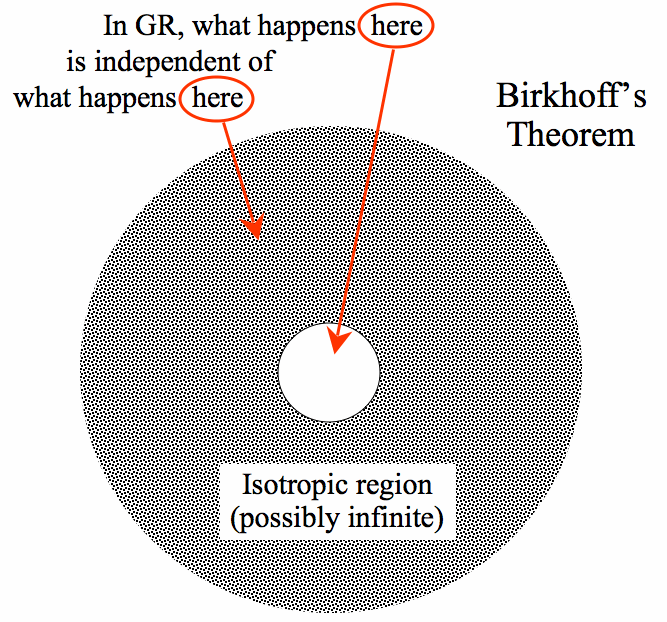
In the General Theory of Relativity, in the case of a uniform distribution of TM (or any mass) in space, only the mass within its orbit affects the motion of an object; homogeneous mass outside the orbit does not affect anything [Birkhoff theorem / approx. transl.]
If there is a sea of TM, permeating all the space where we are with you - the entire solar system - then the outer planets should interact with a slightly larger mass than the inner ones. And if there is a lot of TM here, then there must be a way to detect it. Since we know the mass of the Milky Way, the relative density of normal and dark matter, and we have simulations showing how the density of TM should behave, we can give very good estimates. And after carrying out such calculations, it turns out that about 1013 kg of TM, and the orbit of a planet like Neptune - 10 17 kg.
But these figures are tiny compared to all the other masses! The mass of the Sun is 2 × 10 30 kg, the mass of the Earth is 6 × 10 24 . The masses mentioned by us in the interval 10 13 - 10 17 kg are comparable with the mass of a modest asteroid. Someday, perhaps, we will be able to understand the solar system so precisely that we can detect such tiny differences, but so far we exceed this error by about 100,000 times .

Our galaxy is located inside the huge and scattered halo of TM, so TM must also flow inside the solar system. But its density is extremely small, so it is very difficult to detect it locally.
In other words, TM should be in the Solar System, and influence the movement of the outer planets not as much as the movement of the inner ones, due to the amount of mass in the sphere with the center in the Sun and the radius in distance to the planet. You may be interested in the question whether the interaction of many bodies, namely, TM, planets and the Sun, can lead to the capture of an additional amount of TM. This is an interesting problem, and I wrote a paper on this topic about 10 years ago. My colleagues and I found that the density of TM could increase very much, but only if you do not take into account that the trapped mass, which is very likely, will be thrown back. But even with such an increase, the maximum mass of the TM, after 4.5 billion years (in the graph is purple), is still well below all the observed limitations.

The number of galactic TM, located inside the orbits of planets of different radii of our solar system (blue), and the total number of TM, which should have been captured over the entire lifetime of the solar system, without taking back emissions, as well as the best limit taken from 2013 , by the maximum amount of TM, which in principle can be with us. We have not yet reached the opportunity to check its availability.
In our solar system, there really is a TM, and it should have a real impact on all other particles of matter around it. If there is a cross-interaction between particles of normal and dark matter, then in direct detection experiments it should be possible to detect it directly on Earth. And if not, then the gravitational effects of TM, passing through the solar system, both trapped and free gravitationally, should affect the orbits of the planets. But until our measurements are accurate enough, this gravitational effect will not suffice for any direct detections. So far, we have to look at the Universe outside the solar system in order to observe the effect of TM on space-time.