As the main rival of dark matter died
- Transfer
The only way would be to change the laws of gravity, but the best of our observations dismiss such changes.
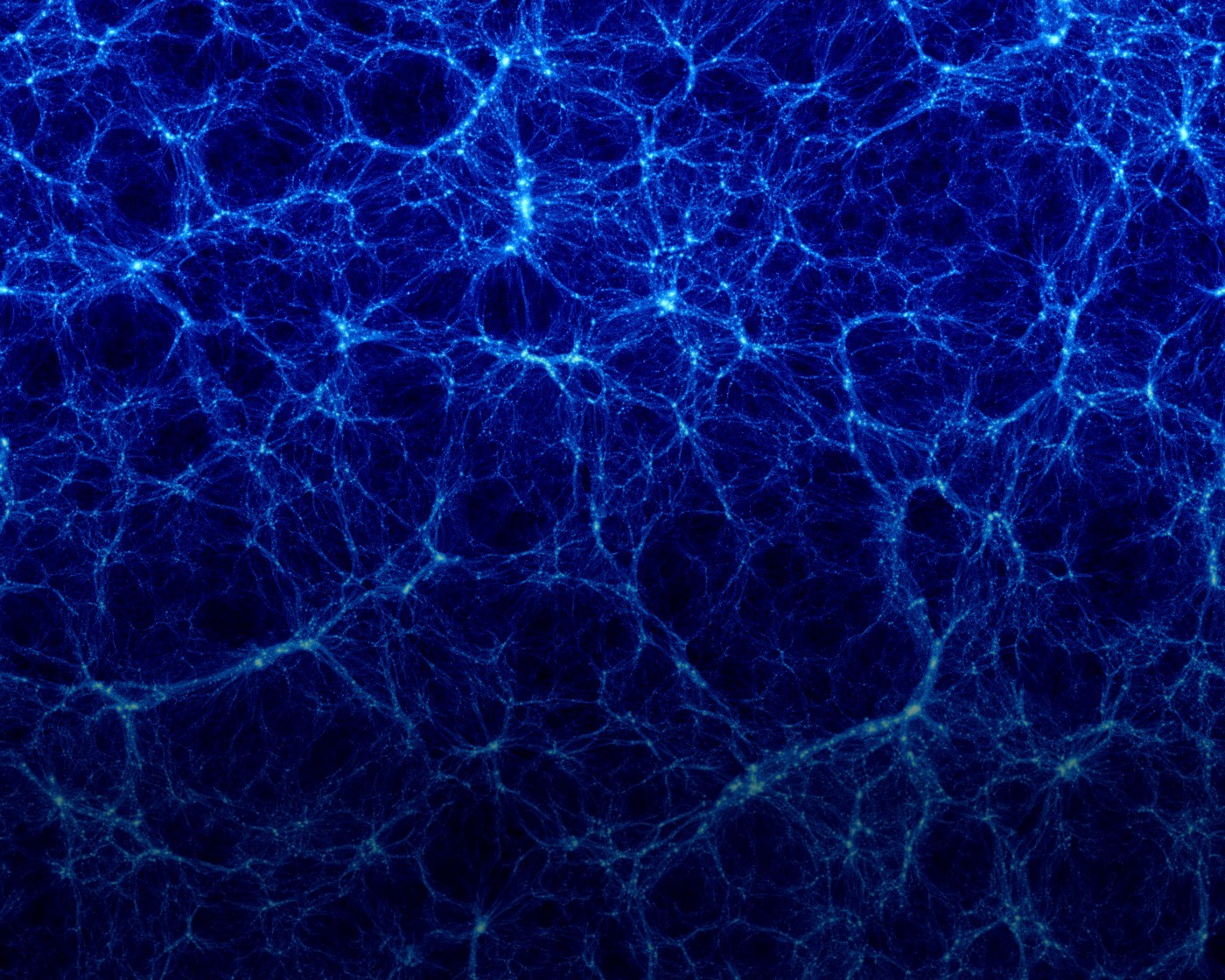
The difference between the expected and the observed has increased over the years, and we are becoming ever more tense to fill this gap.
- Jeremiah P. Ostriker
If you are interested in the cosmos, the Universe and what is in it, you should have heard about dark matter - or at least about the dark matter problem. Let's take a quick look at what you can see if you look at the Universe with the greatest telescope technology that humankind has been able to create.
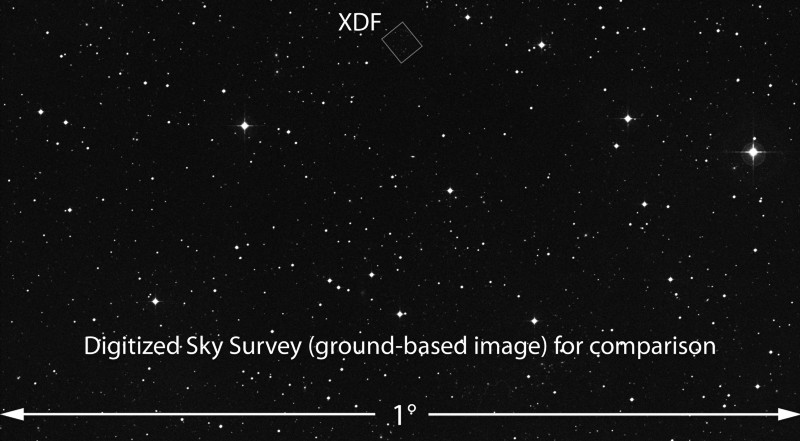
No, not this image. You can see this very well with the armed eye: a small region of space containing a few dull stars in our galaxy, and nothing more.
We considered not only this particular region, but many other similar ones, with the help of incredibly sensitive tools. Even for such a region, devoid of bright stars, galaxies or known clusters and groups, we only need to direct our cameras to it for quite a long time. And if time passes a lot, we will begin to collect photons from incredibly dull and distant sources. This small area called XDF is the result of observation in the Hubble eXtreme Deep Field experiment, a region so small that it would take 32,000,000 to cover the entire night sky of such regions. And that’s what he saw there. Hubble.
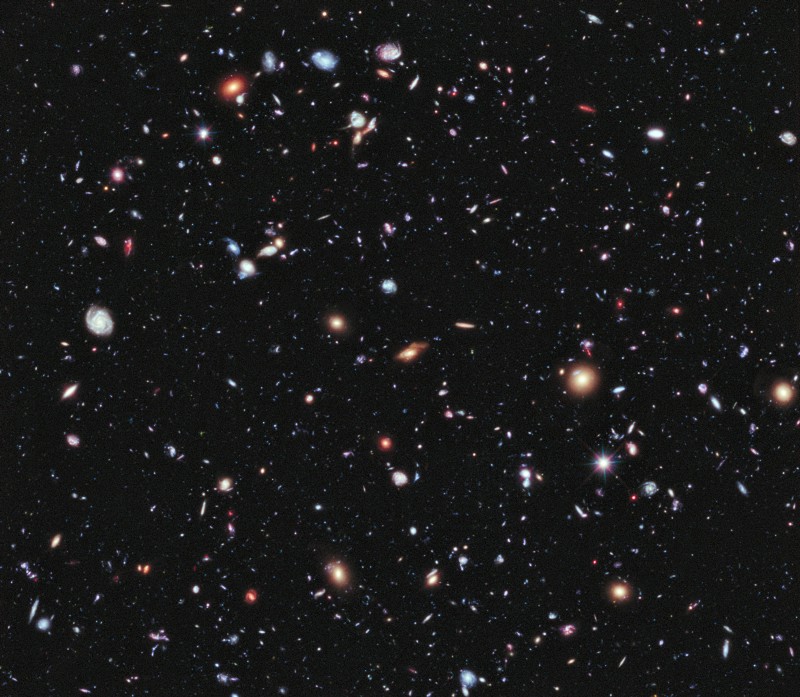
In this picture, 5500 unique galaxies were found, which means that there are at least 200 billion galaxies in the universe. But in spite of the impression made by this number, this is still not the most impressive discovery we made about the Universe when studying a large number and diversity of galaxies, groups and clusters.
Think about what makes these galaxies shine, they are located very close to us or in tens of billions of light years.
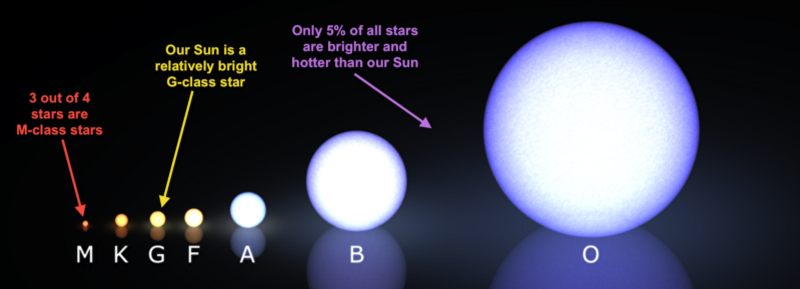
It shines stars in galaxies! Over the past 150 years, one of the main achievements of astronomy and astrophysics has been the understanding of how stars form, live, die and shine during life. When we measure the stellar light from any of the visible galaxies, we can immediately tell what stars are in it and what is their total mass.
Remember this fact as we move forward: the light from galaxies, groups and clusters tells us about the mass of stars contained in the galaxy, group or cluster. But we can measure not only starlight!
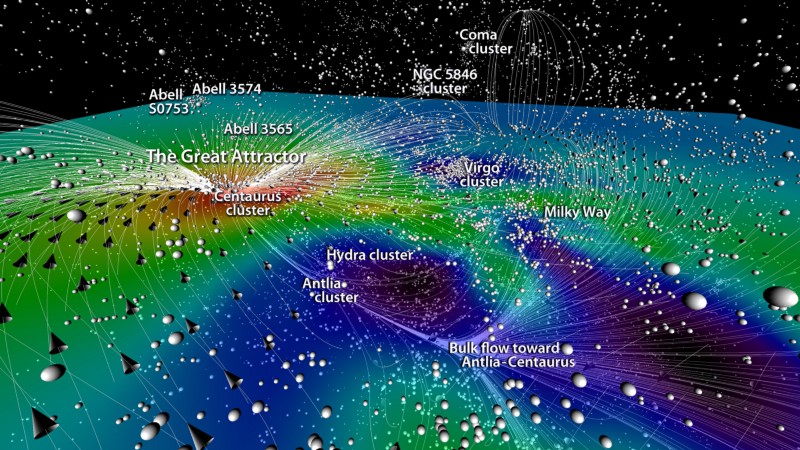
We can measure the movement of galaxies, their speed of rotation, their relative speeds, and so on. It gives us a lot, because on the basis of the laws of gravity, if we measure their speed, we can calculate how much mass and matter must be in them!
Consider: the laws of gravity are universal, which means that they are the same throughout the Universe. The law governing the solar system must be the same as the law governing galaxies. It turns out that we have two different ways to measure the mass of the largest structures in the Universe:
We can measure the stellar light emanating from them, and since we know the principles of the functioning of stars, we can assume how much they contain masses.
We can measure their movement, knowing whether they are related by gravity, and how. Based on gravity, we can assume how much mass is contained in these objects.
And we ask an important question: do these two values agree, and to what extent?
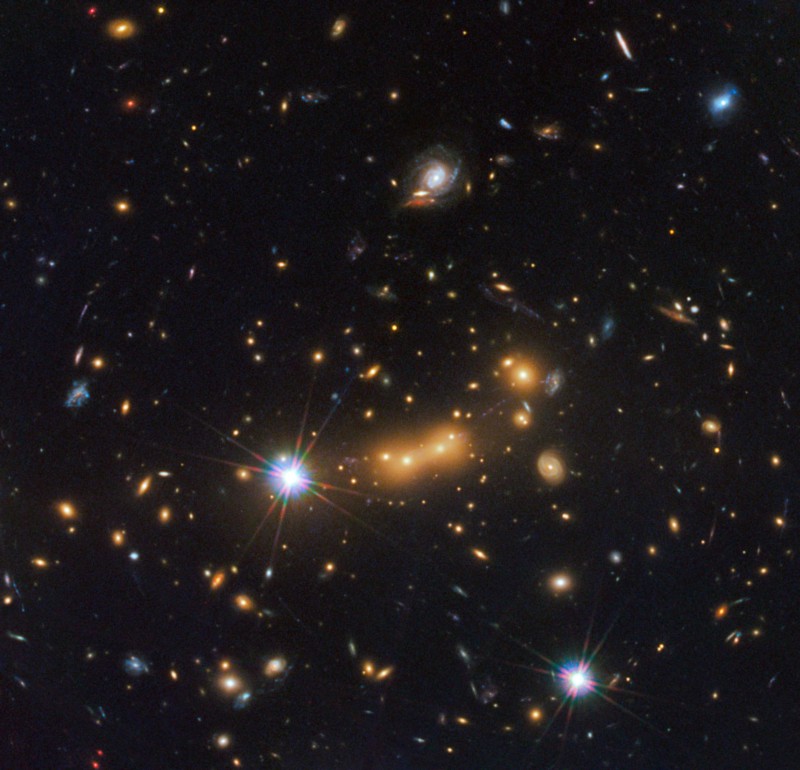
They do not just do not converge, they are not even standing next to! If you count the amount of mass in the stars, you get one number, and if you calculate the amount of mass, based on gravity, you get a number 50 times more. This happens regardless of whether you are studying small galaxies, large galaxies, or groups and clusters of galaxies.
A rather important thing turns out: either 98% of all matter of the Universe is not contained in stars, or our idea of gravity is wrong. Let's consider the first option, since we have a lot of data on it.
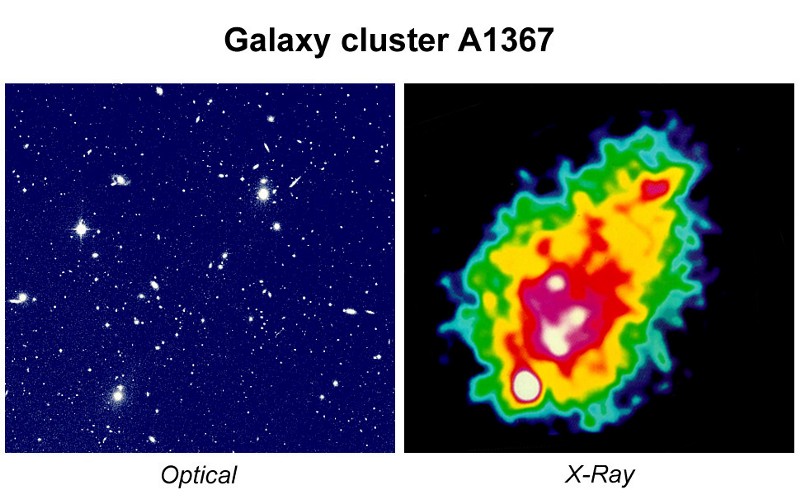
There may be quite a few things besides the stars themselves that make up the mass of galaxies and clusters, including:
- lumps of non-luminous matter, such as planets, moons, small satellites, asteroids, ice blocks, etc .;
- neutral and ionized interstellar gas, dust and plasma;
- black holes;
- remnants of stars like white dwarfs and neutron stars;
- very dim stars and dwarf stars.
The fact is that we measured a large number of such objects and the total amount of normal (consisting of protons, neutrons and electrons) of matter in the Universe from various independent observations, including an abundance of light elements, cosmic microwave background radiation, large-scale structures of the Universe, etc., due to astrophysical observations. We even quite accurately limited the neutrino contribution; and that's what we learned.
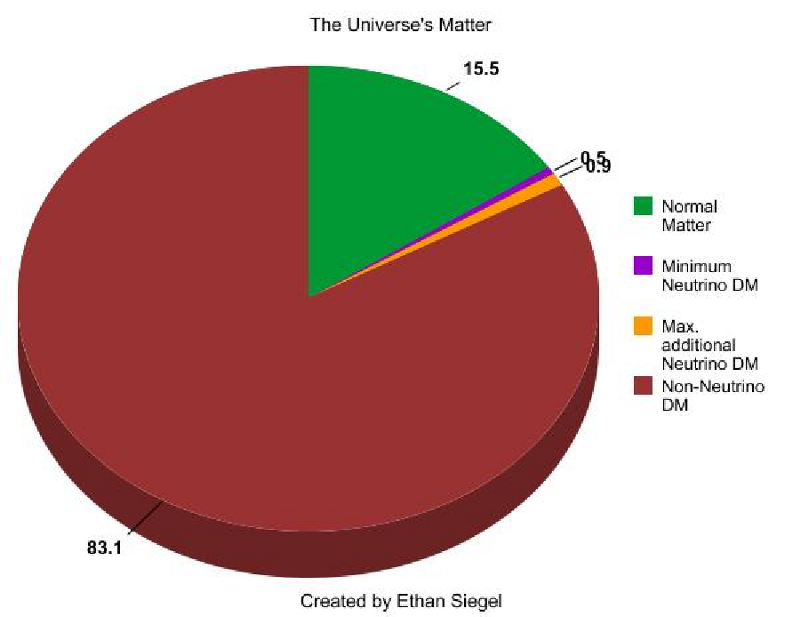
About 15-16% of the total amount of matter in the Universe consists of protons, neutrons and electrons, and most of it is in interstellar (and intergalactic) gas and plasma. There is perhaps another 1% in the form of neutrinos, and the rest is in the mass, which is not composed of particles present in the Standard Model.
This is the problem of dark matter. It is possible that adding some kind of invisible new form of matter is not a solution, but simply the laws of gravity on a large scale do not work that way. Let me tell you a brief history of the dark matter problem and what we learned about it over time.
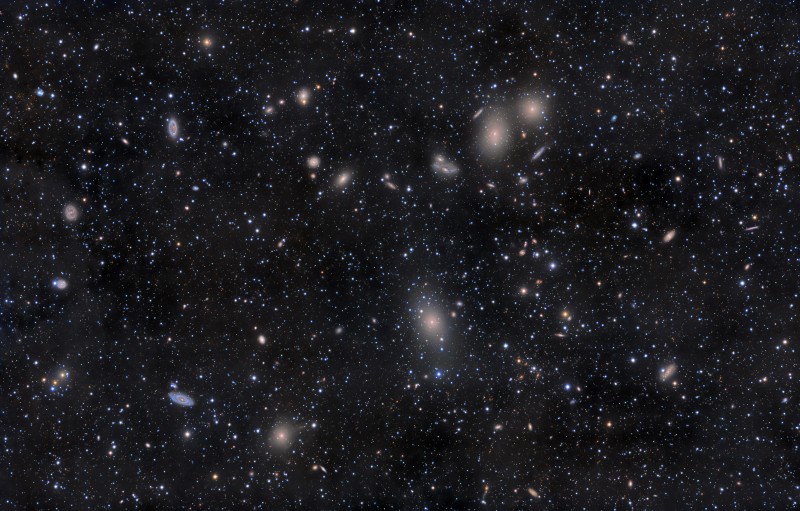
The formation of large-scale structures was initially imagined poorly. But since the 1930s, Fritz Zwicky began to measure starlight emanating from those present in clusters of galaxies, as well as the speed of movement of individual galaxies relative to each other. He noted the great discrepancy, mentioned above, between the mass present in the stars and the mass that must be there in order to connect the clusters with each other.
This work was largely ignored for almost 40 years.
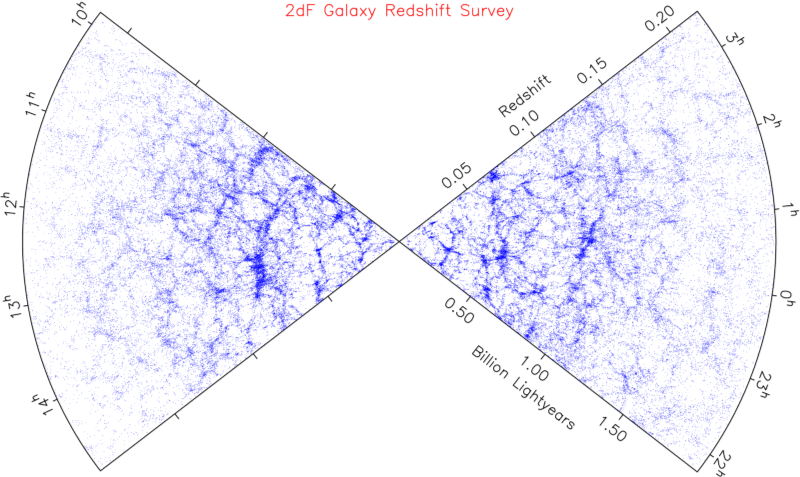
When we began to conduct large-scale cosmological observations in the 1970s, such as PSCz, their results showed that in addition to the problem of the dynamics of Zviki clusters, the structures we observed required an invisible non-barion source of mass in order for these structures to exist. (These results have since been improved by observations such as 2dF, above, and SDSS.)
Also in the 1970s, the work of Vera Rubin, an original and very influential one, drew new attention to the rotation of galaxies and to the dark matter problem that they had clearly demonstrated.
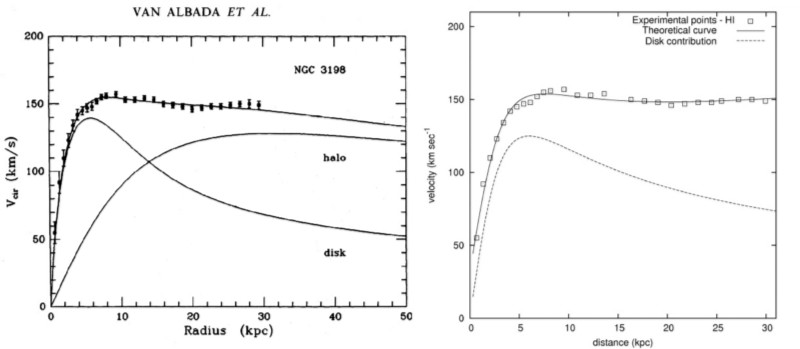
Based on the known data on the law of gravity and observations on the density of normal matter in galaxies, one would expect that, away from the center of a rotating spiral galaxy, stars orbiting around its center would slow down their speed. This would resemble a phenomenon in our solar system, when Mercury has an orbital speed higher, then Venus has a lower speed, Mars has even less, and so on. But in rotating galaxies, instead, it turns out that the speed of rotation of stars remains constant if you move to greater and greater distances from the center, which means that either there is more mass than can be contained in normal matter, or the laws of gravity require rework
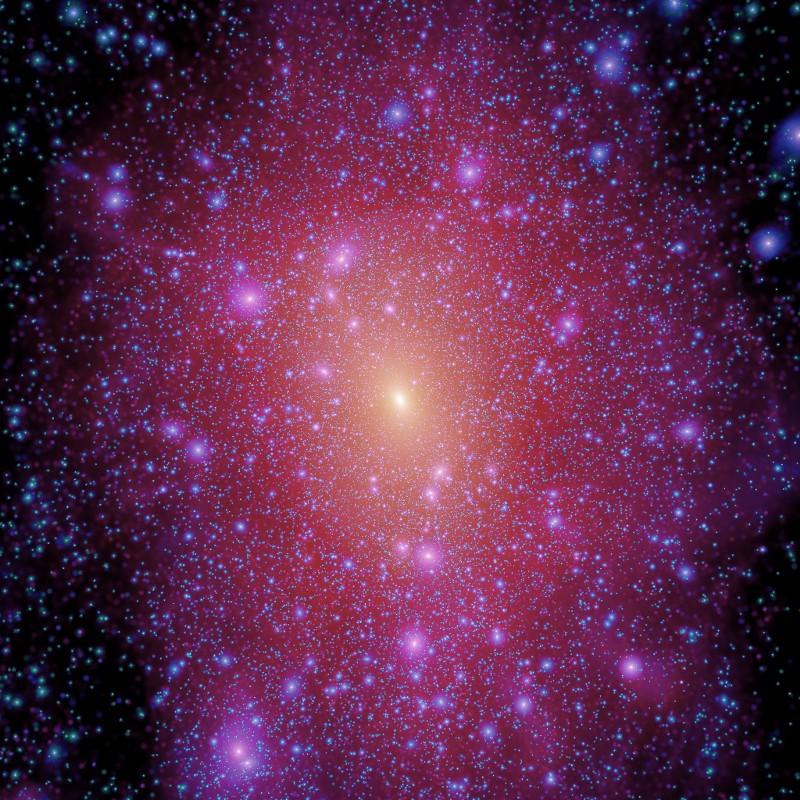
Dark matter was in the lead among the proposed solutions to this problem, but no one knew whether it was baryonic, or not, what temperature characteristics it had, and whether it interacts with itself and with normal matter. We had limitations of what she could not do, and some promising early simulations, but nothing particularly convincing. And then alternatives began to appear.
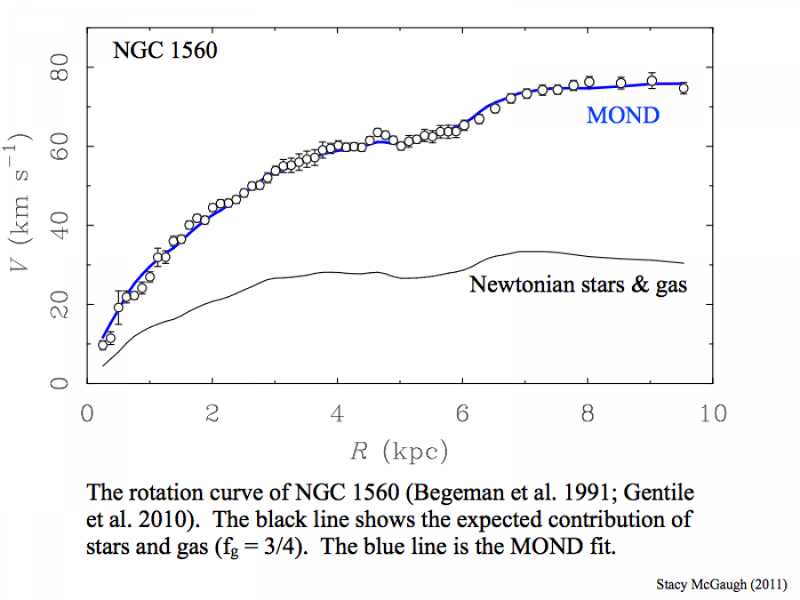
MOND, or MOdified Newtonian Dynamics, a modified Newtonian dynamics, was proposed in the early 1980s as an empirical experimental explanation of the rotation of galaxies. For small structures of galactic scales, it worked well, but did not cope with large scales. She could not explain galactic clusters, large-scale structures and an abundance of light elements, among other things.
And although experts on the dynamics of galaxies seized on MOND, because it explained darkness of galaxies better than dark matter, everyone else was very skeptical, and not in vain.
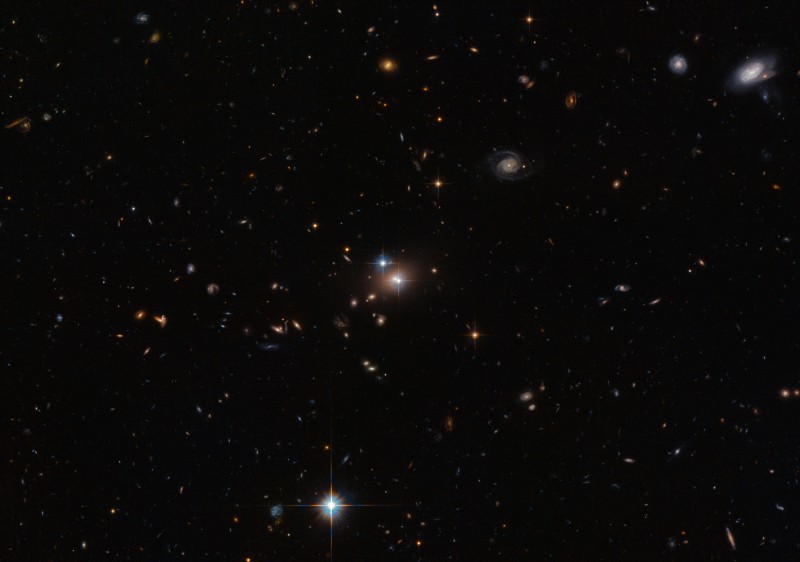
Double quasar, the very first object subjected to gravitational lensing
In addition to failures with structures larger than individual galaxies, the theory turned out to be an unreliable theory of gravity. It was not relativistic, and could not explain the bending of stellar light under the action of mass, the gravitational change of time and redshift, the behavior of binary pulsars or all other relativistic gravitational phenomena, whose conformity with Einstein's predictions was confirmed. The holy grail of MOND - what dark matter followers demanded, and I among them - was a relativistic version that could explain the rotation curves of galaxies along with all the other successes in today's theory of gravity.
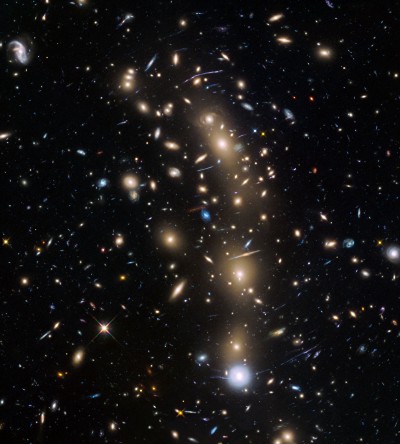
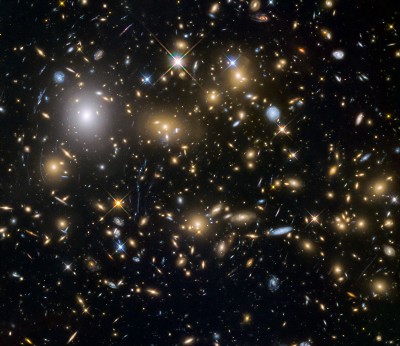
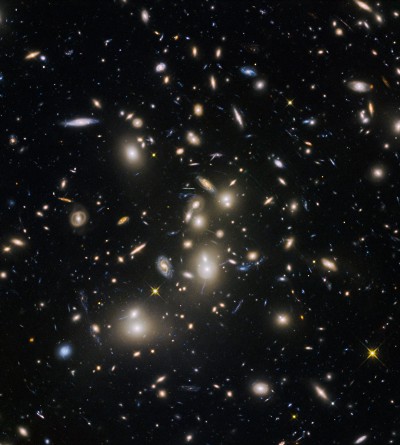
Recently, NASA released a set of images from the telescope. Hubble, who looked further into the past of the Universe due to the phenomenon of gravitational lensing, the consequence of Einstein's theory of gravity. MOND cannot explain this phenomenon in the way it is observed: for none of the lensed galaxies, for a multitude of images, for stretching, or for the magnitude of light bending.
For all this, dark matter is needed, or some invisible source of mass, not consisting of any known Standard Particle Model. But this is not the only evidence we have that refutes alternatives to Einstein's theory or even hypothetical changes that have not yet been discovered, which will make it possible to reproduce MOND.
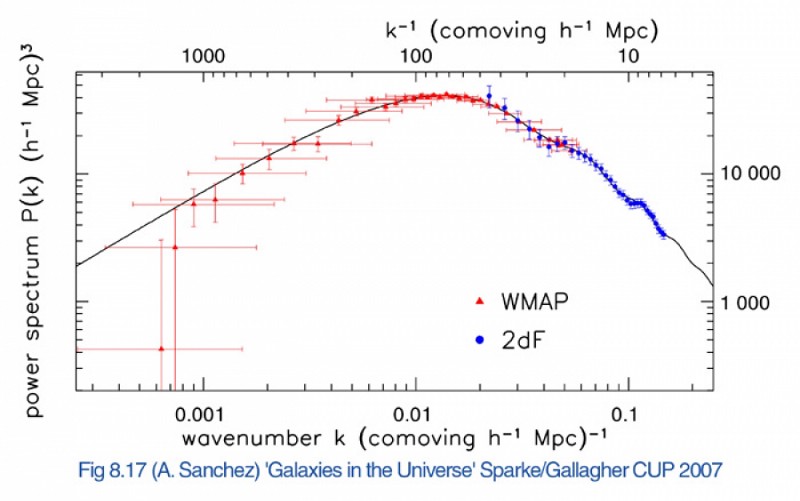
Over the years, dark matter has achieved many cosmological successes. As the understanding of large-scale structures of the Universe from bad to good, and the emergence of an accurate measurement of the power spectrum of matter (above) and fluctuations of the cosmic microwave background radiation (below), improved, it was found that dark matter works fine on a large scale.
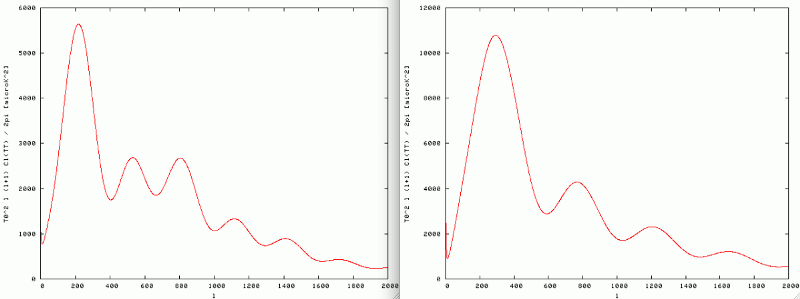
In other words, the new observations - just like the observations made for the Big Bang nucleosynthesis - corresponded to the Universe containing five times more dark matter (non-baryon) than the usual one.
Then in 2005, evidence was discovered. We saw two galactic clusters in the process of collision, and this meant that when confirming the theory of dark matter, we would see how baryonic matter - interstellar and intergalactic gas - would collide and warm up, and dark matter, and the gravitational signal along with it would have to go through and do not slow down. Below you can see the observations in the X-ray range of the Bullet cluster in pink color, which is superimposed by a gravitational lensing depicted in blue.
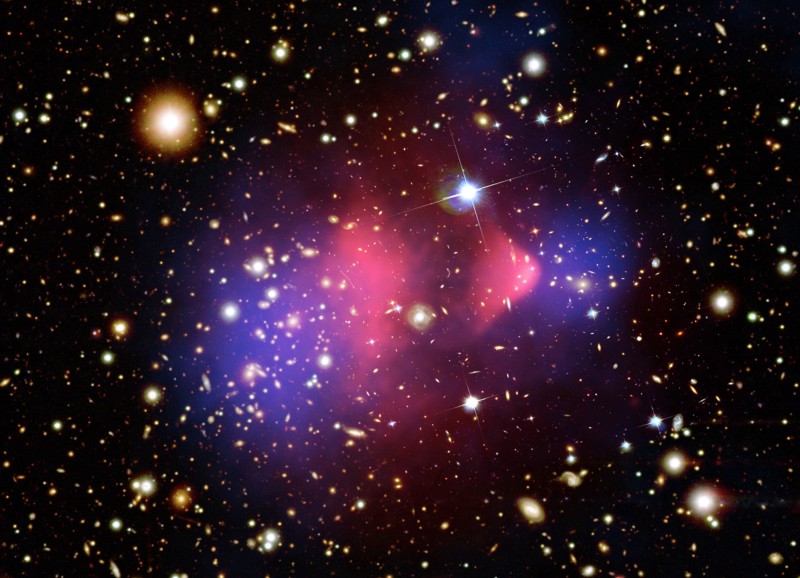
It was a big victory for dark matter and a big challenge to all models of altered gravity: if dark matter does not exist, how could a cluster of "divide the mass and gas" after the collision, but not before it?
However, small scales still present a problem for dark matter; they still do not so well explain the rotation of individual galaxies as MOND. And thanks to its relativistic version, TeVeS, formulated by the late Jacob Bekenstein, now everything looks like MOND has chances.
Gravitational lensing (carried out by ordinary matter) and some relativistic phenomena can be explained with its help and, finally, a clear way has emerged to distinguish between two theories: it was necessary to find an observable phenomenon in which the TeVeS predictions would differ from those of the General Theory of Relativity. Surprisingly, this situation exists in nature.
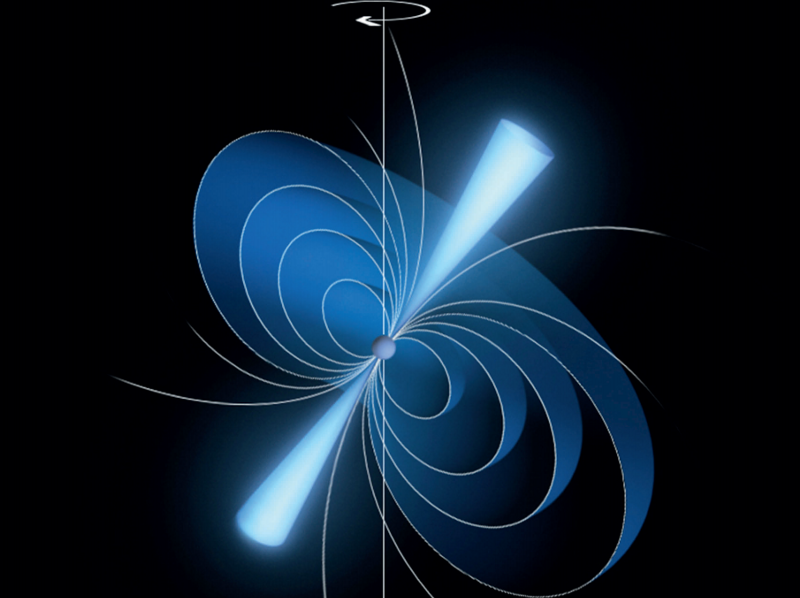
Rotating neutron stars - the remnants of ultramassive stars, turned into a supernova, and left behind the atomic nucleus of the solar mass - tiny objects, only a few kilometers in diameter. Imagine an object 300,000 times heavier than our planet, pressed into one hundred millionth part of the Earth! One can imagine that near these guys the gravitational fields become really tense, and give us one of the most convincing tests in the field of strong fields and the theory of relativity.
It happens so that at a neutron star its axial "rays" are directed straight at us, and they "pulsate" every time the star completes its turn - and such small objects can do this up to 766 times a second! (In the case of such pulsations, neutron stars are called pulsars). In 2004, an even rarer system was discovered: a double pulsar!
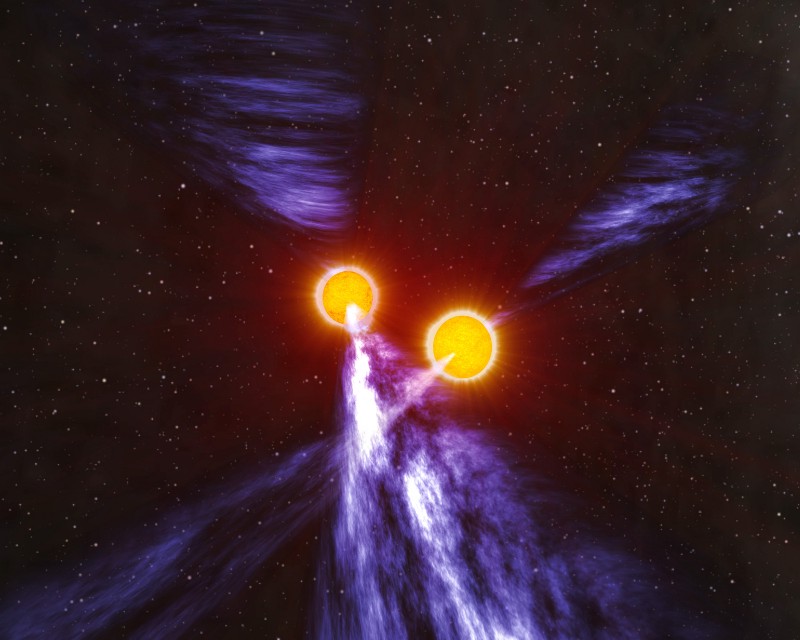
Over the past ten years, observations have been made of the close gravitational dance of this system, and Einstein's GTR has undergone unprecedented testing. You see, when massive bodies move in orbits around each other in very strong gravitational fields, they must emit a very definite amount of gravitational radiation. And although we do not have the technology to directly measure these waves, we have the ability to measure the decrease in orbits due to this radiation! Michael Kramer from the Institute of Astronomy. Max Planck was one of the scientists who worked with this case, and this is what he told about the orbits in this system:
We found that this leads to a decrease in the orbit by 7.12 millimeters per year, with an error of nine thousandths of a millimeter.
And what can TeVeS and GRT say about this observation?
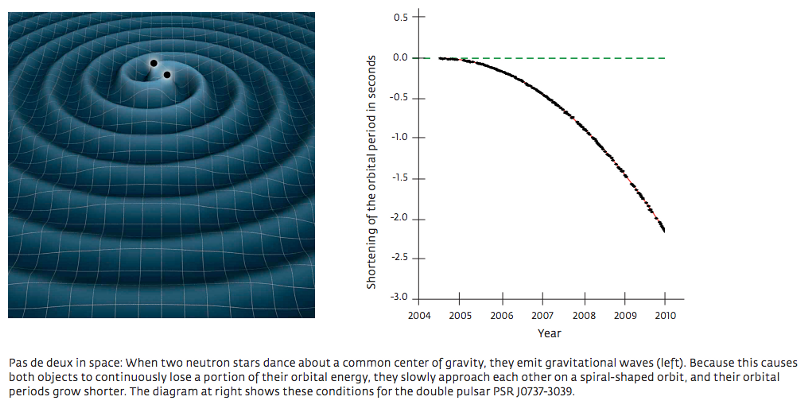
It is consistent with the theory of relativity at 99.95% (with an error of 0.1%), and - prepared - discards all physically meaningful incarnations of Beckenstein's TeVeS. As the scientist Norbert Vex stated with unprecedented brevity:
From our point of view, this refutes TeVeS.
In fact, the most accurate simulation of the formation of structures in history (using GR and dark matter) has recently been published, and it is consistent with all the observations that are compatible with our technical capabilities. Watch this amazing video from Mark Vogelsberger and be amazed!
And, considering all this, that is why the main rival of dark matter dropped out of the competition. He was killed not by dogma, consensus or political will, but by the observations themselves: pulsars, colliding clusters, cosmic microwave radiation, large-scale structures, gravitational lensing. It is still not clear why MOND behaves better on the scale of galaxies, but until it can explain all the other observed phenomena, it will remain the ghost of the theory.