Lipid cold nothing: preventing the crystallization of water at -263 ° C

What is the most on the planet? What is at the top of our needs along with air and food? What, in the opinion of one bearded joke, makes a person related to a cucumber? The answer is simple - water. This chemical compound plays a central role in many macro- and microprocesses: from climate change to the chemical structure of living organisms. H 2 O has a number of chemical and physical properties that are in one way or another used by scientists in various fields. Changing certain parameters leads to the appearance of new properties or a change in old ones. From a young age, many of us know that water under normal conditions boils at 100 ° C and freezes at temperatures below 0 ° C. And then scientists decided to change that.
Today we will meet with you a study in which scientists were able to create water that does not freeze even at -263 ° C. What manipulations were performed to achieve this, what new properties and characteristics did “eternally” liquid water begin to possess, and what is the use of this research? We will look for answers in the report of the research group. Go.
Study basis
The basis of this work is the process of preventing crystallization of water at low temperatures. For this, it is necessary to change the geometry of the water, in which the so-called “confinement”, that is, retention, can help. This mechanism helps prevent low-temperature crystallization of molecules into a hexagonal structure, resulting in amorphous water. Scientists called this physical retention of water at the nanometer level nanoconfiguration. It’s easier said than done, but scientists would not be scientists if they did not have tenacity and a pair of aces up their sleeve. Aces were artificial lipids with cyclopropyl modifications in hydrophobic chains, which exhibit unique liquid crystal behavior at low temperature. These lipids can maintain the amorphous state of water up to -263 ° C.
As a model of closed water in a cellular environment, scientists have chosen confinement inside soft interfaces, such as those formed during the self-organization of surfactants in an aqueous medium. Such a model can help to understand the mechanisms of cell survival at low temperatures.
Researchers draw our attention to the fact that dimensional effects manifest themselves in different phases formed by hydrated monoacylglycerols * at different temperatures and levels of hydration * .
Monoacylglycerols * is a class of glycerides that consist of a glycerol molecule bound to a fatty acid via an ester bond.
Hydration * —addition to water molecules or ions.Monoacylglycerols have a polymorphism, that is, a different crystal structure depending on the conditions: lamellar (L α ), inverse bicontinuous cubic (Q II ), inverse hexagonal (H II ), inverse micellar (L 2 ).
The problem is that this variety of options is lost when temperatures below room temperature are reached, when the general class of lipids crystallizes into a lamellar phase (L c ), in which lipid tails are packed into a long-range crystal lattice. If the temperature drops below zero, then the coexistence of the lamellar phase L c and ice is found at all levels of hydration.
It turns out that you can’t use such lipids? Not really. Lipids can be changed so that their positive properties can be applied, avoiding undesirable restrictions. In previous studies, scientists successfully succeeded in replacing the cis double bond in the middle of the monoolein lipid chain with a cyclopropyl group. As a result of this manipulation, a new lipid will be obtained - monodihydrosterculin (MDS), the phase behavior of which shows the absence of the reverse hexagonal phase and the stability of the Q II D phase at temperatures up to 4 ° C.
Based on the above developments and theories, the scientists presented their own study, which describes a new type of lipid forming mesophases with non-standard properties at low temperature. The most striking property is the ability to retain glassy water at temperatures up to 10 K and at very low cooling rates.
Lipid polymorphism
To begin with, scientists explain certain nuances regarding lipid polymorphism. In nature, at the moment there is a very limited number of lipids that can form Q II phases.
Lipid chains provide the fundamental elements of all mesophases. Their molecular structure, a certain length, curvature, position and degree of unsaturation affect the final mesophase * .
Mesophase * - the state of a substance between a liquid and a solid.If we replace the cis-double bond of monoacylglycerols with a cis-cyclopropyl fragment, then the curvature of the chain and the length of the lipids will be reduced to the original, but the fractional compaction and lateral tension of the tails will be significantly changed. And to change the stiffness of the lipid tail, it is necessary to change the number and position of cyclopropyl groups, as well as the length and curvature of the hydrophobic chains.
During the study, scientists synthesized three lipids (the structures are shown in 1a ): monodihydrosterulin (MDS), cyclopropane monolactobacillin lipid (MLB) - an analogue of monovaccine (MV) and DCPML - monolinolein (ML).

Image No. 1
The graphs above show the results of small-angle X-ray scattering (MRI): phase diagram of the composition and temperature of the MLB sample ( 1b), phase diagram of the composition and temperature of the DCPML sample ( 1c ).
Judging by the observations, hydrated MLB has a transition sequence, as in classical monoacylglycerols ( 1b ), in which L α , Q II G and Q II D are observed with increasing hydration level. Unlike MDS, the H II phase is present in MLB at high temperature.
It was possible to find out that the phase H II and the cubic phase Q II D remain stable in excess of water. This observation made it possible to determine the boundary degree of hydration for both phases by analyzing the lattice parameters at each hydration level.
In the case of DCPML lipid, scientists noticed an unusual phenomenon - the formation of a cubic phase Q II G at 22 ° C with a water content of only 5% ( 1s ).
Previous studies have shown that the formation of H II with pure hydrated monoacylglycerols is possible only at high temperatures (above room temperature). Stable H II phases at room and physiological temperatures (≈ 36.6 ° C) require the use of hydrophobic molecules or the presence of an ether rather than an ester bond.
The formation of the H II phase at room temperature suggests a shift in the DCPML phase diagram to lower temperatures and hydration, which was confirmed in this study.
A DCPML sample with 12.5% water was first gradually cooled to -20 ° C, and then again heated to 22 ° C. At the end of each cooling and heating stage, the system was balanced, and MPP data were also collected ( 2a ).

Image No. 2
The phase transition from L α to Q II G occurs in the temperature range −15 ... −10 ° C during both heating and cooling procedures. It was also revealed the formation of a new stable lipid cubic phase at low temperatures. When heated, the radius of the water channel of the Q II G phase decreases - from 8.4 Å at -10 ° C to 7.8 Å at 22 ° C.
As a result, scientists got an absolutely stable cubic phase Q II Gat subzero temperatures. This observation contradicts the generally accepted facts that lipids (for example, monoolein) form cubic phases, which crystallize into a lamellar crystalline phase and ice at temperatures below 0 ° C.
Properties and behavior of water
The liquid crystal nature of DCPML at low temperatures indicates the non-standard characteristics of water contained in nanochannels. The size of the water areas (plates or channels) can be manipulated by changing the water / lipid ratio. Melting transitions were studied using differential scanning calorimetry (DSC) measurements of mesophases at various hydration levels ( 2b ).
DCPML samples underwent cyclic heat treatment (heating - cooling - heating) from −70 ° C to 60 ° C with a scan rate of 5 ° C per minute. What we see in graph 2bwas obtained during the second heating process. At a water concentration of 20 and 25% in the sample, a peak of ice melting is visible at 0 ° C, which is typical for pure water (without the addition of lipids). If hydration increases, then this peak begins to decrease (15% of water), and then completely disappears (5% and 10% of water). The conclusion is quite obvious - confinement in the phases L α and Q II G at a low level of hydration prevents the crystallization of water at the considered cooling rate.
Also, in graph 2b , small peaks can be seen at high temperatures, which correspond to transitions between different geometries and correspond to the results of MPP ( 1с) Differences in the transition temperature by several degrees can be explained by different heating rates and, accordingly, different balancing times. Of course, one should not forget about the error (1.5%), which depends on the composition of different samples.
Scientists note that ice formation is present in ML at temperatures down to -60 ° C, while the amorphous state is preserved in DCPML. This suggests that confinement alone cannot prevent crystallization, but works in conjunction with the liquid crystal behavior of lipids to achieve this.
Next, the samples were cooled to -263 ° C at a rate of 0.1 ° C per minute, balanced and then heated at the same speed. In images 2c and 2dwe see the results of FWS measurements during heating, which show the absence of a first order transition in low water DCPML. Scientists have chosen a sample with a water content of 7.5% in order to ensure a uniform geometry in the entire temperature range below zero.
The FWS profiles in graphs 2c and 2d do not show any jumps in the region of 0 ° C, although an increase in mobility is observed at a temperature of about -50 ° C. Scientists note that the mesophase obtained from commercial ML instead of DCPML with the same topology and water content shows melting at a temperature of about -10 ° C (peaks in the insets in 2s and 2d) DCPML at 15% water in the sample also shows a jump, which corresponds to melting ice at a temperature of about -10 ° C. However, judging by the DSC data, the transition intensity in this case is much lower, that is, only part of the water is involved in the formation of ice. And the absence of a jump for the lipid-lipid transition confirms the absence of the crystalline phase L c in DCPML.
Experiments using wide-angle x-ray diffraction (WAXS) at low temperatures showed the hexagonal structure of ice in samples with hydration of 20% and 25 ( 2e ), as well as the absence of crystallization in the WAXS region for other samples. The observation data once again confirm the liquid crystal nature of the lamellar phase (L α) and the absence of crystalline ice at low hydration.
Finally, scientists also used NMR spectroscopy to study water mobility and phase behavior ( 2f ). For a sample with 7.5% water, the detection limit was reached at 0 ° C, which indicates a diffusion coefficient of less than 10 -11 m 2 / s. And for a sample with 10% diffusion was observed to -11 ° C.
Thus, the quasilinear temperature dependence of diffusion confirms the liquid state of water in the considered temperature range, and additional information obtained from FWS and DSC analyzes confirms the transition of water from liquid to glassy at low temperatures.

Image No. 3
Scientists combined all the data collected and were able to compose a phase diagram of the water contained in the DCPML mesophases ( 3a ).
It is worth noting that the observed processes and characteristics are closely related to the features that distinguish DCPML from all other known monoacylglycerols, namely, the general shift of phase transitions towards lower temperatures and hydration, as well as the absence of L c even at extremely low temperatures.
Image 3b shows the results of MPR measurements of lipid geometry, superimposed on top of the phase diagram of water ( 3a ). During hydration, the reverse transition L α → Q II G → L α is observedin the temperature range from -10 ° C to 0 ° C. Interestingly, the presence of liquid water at subzero temperatures due to the stability of the cubic phase Q II G . And with a decrease in hydration during cooling, the combination of lipid disorder and geometric phase restriction L α prevents ice formation at any temperature.
If the degree of hydration is increased, then the formation of hexagonal ice will be observed. Observations showed that with hydration of 20% and cooling of the sample to -30 ° C, the Q II G phase is stable for several hours, with no ice being detected. Transition to the phase L αoccurs after an incubation of the sample for 1 hour at a temperature of -40 ° C, and here ice formation is already observed. When heated from −40 ° C, the L α phase remains stable up to 0 ° C. Between -40 ... -20 ° C, the lattice parameter α shows the expected decrease (from 39.2 Å to 38.4 Å), typical of mesophases. But already in the range of -20 ... -10 ° C the situation is the opposite: an increase from 38.4 Å to 39.2 Å, which is usually associated with increased hydration of the lipid bilayer.
In addition to all the observations, measurements and various scanning techniques, the scientists also used molecular dynamics modeling to confirm the results of the study.
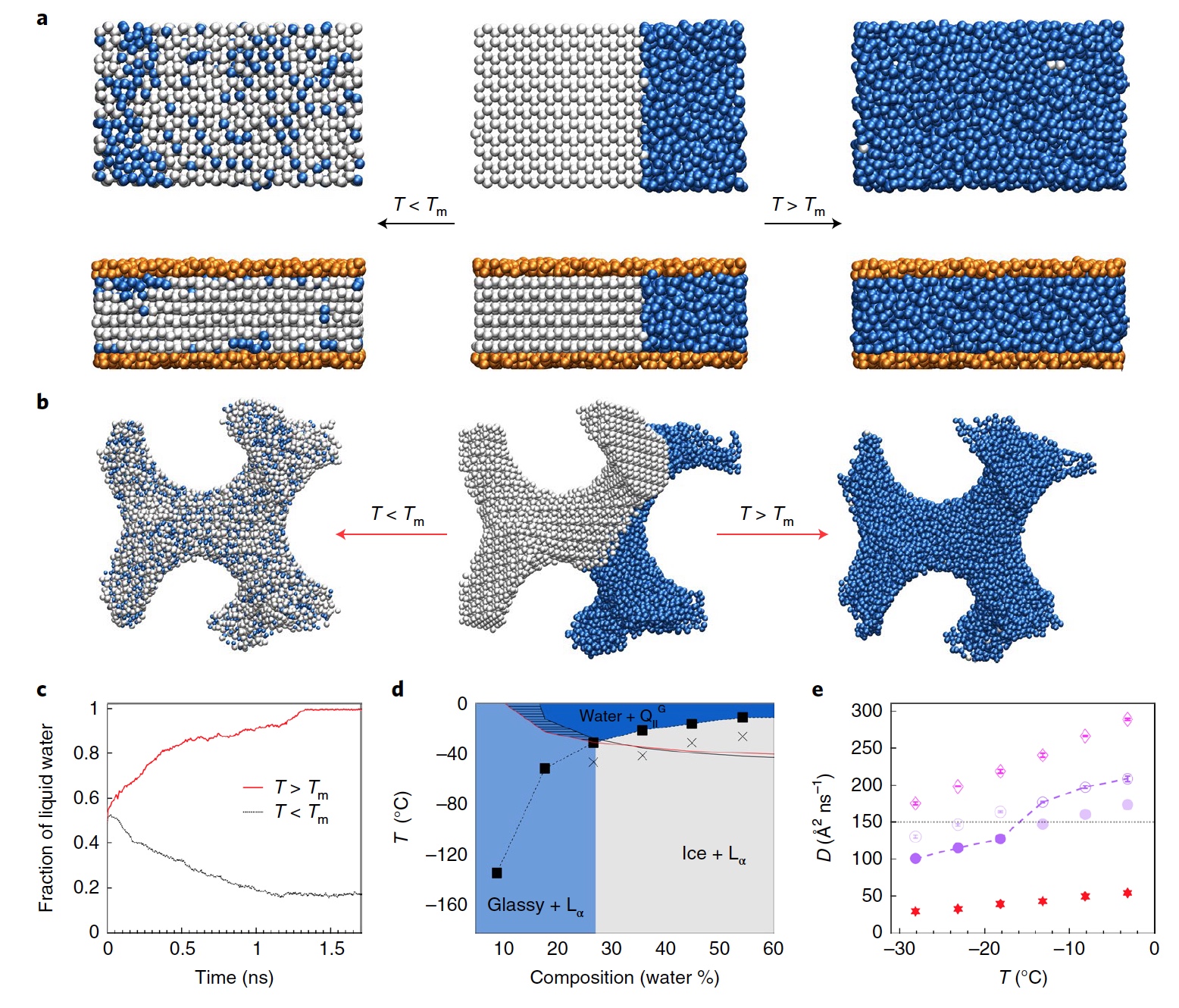
Image No. 4
Researchers are well aware that the results of such a simulation strongly depend on a whole set of variables: the interaction between water and lipid molecules, the lipid-lipid transition, the transition threshold to the glassy state, etc. However, they argue that the results of their modeling are fully consistent with observations.
Figure 4a shows a molecular dynamics model for the melting temperature of the plate mesophase at 54.3% hydration. In the center we see the starting configuration, which is partially filled with ice (white spheres) and liquid water (blue spheres). The final configuration below the melting point is shown on the left. And on the right - above the melting point. The upper row is a system without lipids, the lower one with lipids (orange spheres). Pictures 4bthis is a presentation of water enclosed in a cubic phase Q II G with hydration of 54.3%, for the initial (in the center) and final configuration below (left) and above (right) melting point. In turn, graph 4c shows the temporal evolution of water above (red line) and below (black line) melting point.
Researchers note that with low hydration, the system follows “standard” behavior, that is, it moves from cubic to lamellar structure ( 4d ). Upon cooling, the Q II G phase passes to L α , showing a sudden decrease in water mobility ( 4e) Less mobility means the system needs more time to balance. In this mode, the cooling process crosses the melting line after diffusion is already difficult, that is, before the crystallization of water, as a result of which we observe glassy water.
For a more detailed acquaintance with the nuances of the study, I strongly recommend that you look into the report of scientists .
Epilogue
Scientists are accustomed to expand the boundaries of our worldview, understanding various processes and phenomena. Some studies are the starting point for future technologies and new discoveries, and some are just food for curiosity. Today's belongs to the first category. Understanding the behavior of the two most important elements of life (water and lipids) at extremely low temperatures can help develop new methods for the diagnosis and analysis of biomaterials that are difficult or even impossible to analyze at room temperatures due to their instability. Scientists also talk about the prospect of changes in living cells, that is, their modification to normal functioning in very low temperatures. In other words, if we consider hypopsychological planets (−50 ° C and below) and psychroplanes (−50 to 0 ° C) as possible options for colonization,
Thank you for your attention, remain curious and have a good working week, guys!
Thank you for staying with us. Do you like our articles? Want to see more interesting materials? Support us by placing an order or recommending it to your friends, a 30% discount for Habr users on a unique analogue of entry-level servers that we invented for you: The whole truth about VPS (KVM) E5-2650 v4 (6 Cores) 10GB DDR4 240GB SSD 1Gbps from $ 20 or how to divide the server? (options are available with RAID1 and RAID10, up to 24 cores and up to 40GB DDR4).
VPS (KVM) E5-2650 v4 (6 Cores) 10GB DDR4 240GB SSD 1Gbps until the summer for free when paying for a period of six months, you can order here .
Dell R730xd 2 times cheaper? Only here2 x Intel Dodeca-Core Xeon E5-2650v4 128GB DDR4 6x480GB SSD 1Gbps 100 TV from $ 249 in the Netherlands and the USA! Read about How to Build Infrastructure Bldg. class using Dell R730xd E5-2650 v4 servers costing 9,000 euros for a penny?