Radiation: units
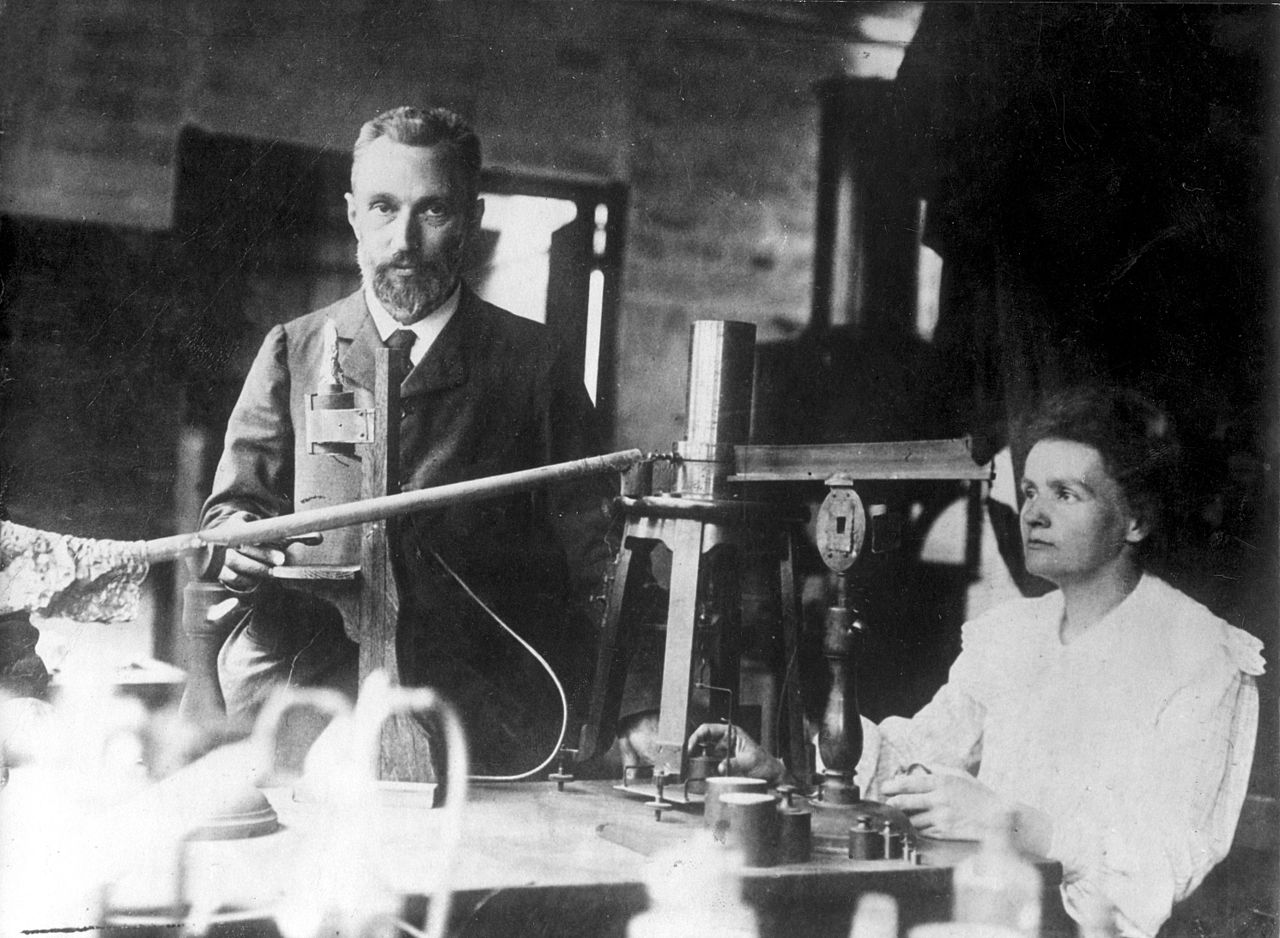
With almost every conversation about radioactivity with a layman, it turns out that the interlocutor has, to one degree or another, a vague idea of units of measure. So when I published an article about the radiochemical laboratory, one of the readers complained to me in PM that he had from a lot of units found in books and articles on radioactivity - X-rays, rems, rems, glad, greys, sievert, curies, becquerels and even gram equivalents of radium - my head is spinning and asked to write about it. I fulfill his request.
Yes, on KDPV - spouses Maria Sklodovskaya-Curie and Pierre Curie.
A bit of history
In 1895, Wilhelm Konrad Roentgen discovered radiation that had amazing properties: acting like light on photographic plates, and exciting the glow of fluorescent screens, it easily penetrated through opaque obstacles. Not much time passed, it turned out that the source of such radiation is not only a working Crookes tube, as in the X-ray experiments, but also substances containing uranium, which, in addition, emit this radiation continuously, invariably and without any supply of energy from the outside. This was followed literally by an avalanche of discoveries. The discovery of radium, polonium, and then a whole bunch of new radioactive elements, the establishment of a connection between radioactive decay and the conversion of one element to another, the first nuclear reactions carried out ... In general, Becquerel's surprisingly simple experiment with uranium salt on a photographic plate wrapped in black paper literally printed a “pot-not-boil” of new knowledge. The conversation about these discoveries is the topic of another article (and not one), and now I’ll just say that already then, in the first months and years of this “radium boom”, measurements could not be dispensed with.
The first measuring device for determining the intensity of ionizing radiation was an ordinary electroscope or electrometer, which was discharged under the influence of radiation, and the speed of this discharge was proportional to its intensity. And the first standard was ...
Milligram of radium ampoule as a measure of radioactivity
This ampoule was not only the first standard for the calibration of electrometers and ionization chambers - it was a measure of the amount of radioactivity. An amazing property of radium was the exceptional constancy of its radiation: its intensity depended only on the amount of radium. Therefore, having once taken a sample of 1 mg of radium and sealed it in a platinum ampoule, it became possible to never weigh the radium again. By comparing the intensity of gamma radiation from a reference ampoule and a sample placed in an ampoule with the same wall thickness, it was possible to determine the amount of radium in it with high accuracy. So the ampoules with radium took their rightful place in the chambers of weights and measures next to the standards of meter, kilogram
Strictly speaking, the source of gamma radiation is not radium. And it is precisely with this that the sealed ampoule was the standard . The fact is that radium-226 does not emit gamma rays during decay. It emits an alpha particle, turning into radon-222, which was then called the emanation of radium . The latter, being also alpha-active, then undergoes a series of decays with the emission of alpha and beta particles, some of which are accompanied by gamma radiation. Radon has nowhere to go from a sealed ampoule, and secular equilibrium is established between radium and its radioactive decay products : how much radon (and each subsequent member of the radioactive series) has formed, so much decays.
When comparing the radioactivity of other subsequently discovered elements with radiation, they began to use such a unit as the milligram equivalent of radium , equal to the amount of radioactive substance that gives the same gamma radiation intensity as milligrams of radium at the same distance.
The milligram equivalent of radium, as a unit of radioactivity, has the obvious drawback that gamma radiation, generally speaking, is a kind of side effect of radioactive decay. In many cases, it is either absent or not in every act of decay. Therefore, we switched from comparing gamma-radiation intensities to the concept of activity as a measure of the number of decay events in a preparation per unit time . The same ampoule with radium remained the standard, and from here a unit appearedcurie , defined as the activity of a radioactive substance in which as many atoms decay per unit time (namely,
The curie unit is now considered obsolete, like all non-system units. In the SI system, becquerel replaces it - this is the activity of the drug, in which on average one decay per second occurs. Thus, 1 Ki =
Electrometer and exposure dose
The first device for measuring the intensity of radioactive radiation, as I said, was an electrometer, which was discharged under the influence of radium rays. He became the forerunner of the ionization chamber - a chamber with two oppositely charged electrodes, which made it possible to determine the amount of ions formed in the air filling the chamber. These ions in an electric field inside the ionization chamber begin to move towards the electrodes and, having reached them, discharge them. The magnitude of the decrease in the charge of the electrodes can determine the number of pairs of ions that are formed in the air under the influence of radiation. And by measuring the current flowing through the chamber in the circuit of an external voltage source, it is possible to determine the number of ion pairs generated in the chamber per unit time, proportional to the radiation intensity.
The value thus measured was called the exposure dose of the radiation. And the unit of measurement was x-ray . At an exposure dose of 1 x-ray in one cubic centimeter of dry air, one unit of HSE is formed (
There is no special unit of exposure dose in the SI system and the unit is pendant per kilogram. 1 C / kg = 3875.97 R. However, at present this unit is used extremely rarely due to the rejection of the very concept of exposure dose. The reason for this failure is that this rather easily measurable quantity is of little use for practical application. Usually, we are not interested in how many ions formed in the air, but in the action that irradiated the substance or living tissue.
Absorbed dose
The idea to consider the energy absorbed in this substance as a measure of the effect of radioactive radiation on a substance is quite obvious. This is the absorbed dose , the measure of which is the radiation energy absorbed by a unit mass of the substance. The unit of measurement of the absorbed dose in SI is gray : 1 Gy = 1 J / kg. Previously, another unit was used - glad . 1 rad = 100 erg / g = 0.01 Gy. At an exposure dose of 1 P, the absorbed dose in the air is 0.88 rad. In most cases, these 0.88 are rounded to unity, equating rad to x-ray (although in fact they are different physical quantities), and gray (and sievert, which is described below) to 100 x-rays.
But the dose in various substances at the same exposure dose will be different depending on the type and energy of radiation and the properties of the absorber. It is for this reason that the concept of exposure dose has now been abandoned. In practice, it is much more correct to measure not the exposure dose, but to take a detector whose average atomic number is equal to the average atomic number of biological tissue (in this case we speak of a tissue-equivalent detector ) and measure the absorbed dose in it. Then, with a certain degree of accuracy, it can be assumed that the absorbed dose in the detector will be equal to the absorbed dose in biological tissue.
All sorts of different doses
But it turns out that different types of radioactive radiation act on living tissue differently. Alpha radiation, protons and neutrons at the same absorbed dose cause it much more harm than gamma radiation and beta particles. In this regard, along with the absorbed dose, another type of dose arises - the equivalent dose . It is equal to the dose of gamma radiation, which causes the same biological effect as the dose of this radiation.
The unit of equivalent dose is sievert . The old unit of equivalent dose is the biological equivalent of x-ray or rem , in English REM(sometimes in the translated literature and radiologists you can find the unit "rem" - this is the same rem). 1 Sv = 100 rem.
In order to translate the absorbed dose into the equivalent, you need to multiply the absorbed dose by the so-called quality factor . This coefficient for photons, electrons and muons is equal to unity, for alpha particles it is assumed to be equal to 20, for protons according to various sources it is from 2 to 5, and for neutrons it is very dependent on energy, reaching 20 in the energy range from 100 keV to 2 MeV ( see picture).

In addition to the equivalent, an effective dose is also considered. It takes into account not only a different degree of harmfulness of radiation, but also a different degree of harmfulness of irradiation of one or another part of the body or organ when irradiating not only the whole body, but its part. Weighing factors are attributed to each tissue and organ in such a way that the sum is equal to one. With uniform exposure to the whole body, the effective dose is equivalent. It is measured in the same units as the equivalent.
I will stop here: I won’t confuse you and tell you what kerma, the ambient dose equivalent, and many more things are.
And how does it all measure?
To measure the exposure dose, as I said, you need to take a certain amount of air, collect the ions formed in it and determine their amount, which can be successfully solved using an ionization chamber. It is on the basis of ionization chambers that the bulk of the accumulative dosimeters of the "pencil" type were made.
And in order to measure the absorbed dose, you have to measure the amount of energy released in the substance. And here lies the main difficulty. Directly measuring this energy is very difficult, since in most cases it is very small. One gray (and this is a serious dose, already causing radiation sickness) is just a joule per kilogram. If we try to measure this dose, for example, calorimetrically - by temperature change, then, for example, aluminum will heat up just a little more than a thousandth of a degree.
Therefore, all methods of measuring the absorbed dose or its power are indirect. They consist in the fact that we observe a certain process caused by irradiation and requiring energy expenditures and assume that the “output” of this process will linearly depend on the energy contribution of the absorbed radiation to it.
The primary act of interaction of ionizing radiation with matter is almost always ionization itself. A gamma-ray quantum or other particle emitted by a radioactive substance usually has an energy that is much higher than the energy needed to tear an electron out of an atom. Therefore, it does not end with one act of ionization. Along the entire trajectory of the particle, free electrons and positively charged ions are generated in the substance, the energies of which usually exceed the ionization energy themselves, which leads to the development of a whole cascade of processes for the formation of free electrons and ions, until their energy is comparable with the chemical bond energy , with the first ionization energies, etc. And already these electrons and ions directly carry out that effect on matter, which is characteristic of ionizing rays: they excite luminescence, initiate chemical reactions, destroy biological structures, and become carriers of electric current. Both their quantity and total energy are proportional to the absorbed dose (strictly speaking - minus the energy of the electrons flying out of the substance), and they already “know nothing” about what gave rise to them.
Historically, one of the first dosimeters was an ordinary film wrapped in a lightproof material. The degree of its blackening after manifestation approximately depends on the absorbed dose as well as on exposure to ordinary visible light: there is a region of linear dependence limited by bending in the low-dose region and saturation (with subsequent solarization - decrease in density) in the high-dose region. The film is a cheap and rather sensitive, but not very reliable dosimeter, since small deviations in the treatment regimes can give noticeable dose determination errors. Photographic film is one of the first representatives of the family of chemical dosimeters in which the dose value is determined by the amount of a substance formed or consumed during the reaction: colored, paramagnetic, or possessing another easily measurable property. It can be a solution in an ampoule that darkens or stains under the influence of radiation (for example, due to the oxidation of iron (II) to iron (III) with the subsequent formation of brightly colored red thiocyanate), glass or a crystal in which the so-called radiation defects absorbing light. Chemical dosimeters make it possible to determine the radiation dose with high accuracy and within a very wide range - from those that do not cause a person special harm to those that kill him in one minute. But, as a rule, they do not allow measuring the dose rate. absorbing light. Chemical dosimeters make it possible to determine the radiation dose with high accuracy and within a very wide range - from those that do not cause a person special harm to those that kill him in one minute. But, as a rule, they do not allow measuring the dose rate. absorbing light. Chemical dosimeters make it possible to determine the radiation dose with high accuracy and within a very wide range - from those that do not cause a person special harm to those that kill him in one minute. But, as a rule, they do not allow measuring the dose rate.
Luminescence makes it possible to detect even the act of absorption of a single particle or gamma ray, which leads to the appearance of a short light flash in the detector material - scintillation. This principle is based on the action of scintillation detectors, which make it possible to measure even very weak radiation fluxes that are tens and hundreds of times weaker than the natural radiation background. The scintillation radiation sensor, unlike chemical detectors, allows you to determine the power of the dose absorbed by the detector in real time. Of course, in order to obtain the dose value, or dose rate, it is necessary not only to count the number of pulses, but to summarize and integrate the light emitted by the scintillator.
A special kind of such detectors are the so-called thermoluminescent detectors. They use luminescent material, which, instead of flashing every particle with a flash of light, retains the free charges formed by it in the form of long-existing charged lattice defects. When heated, these defects “heal”, and the released electrons and holes recombine, transferring energy to the luminescence centers. And integrating the light pulse that occurs when the thermoluminophore is heated, we will determine the dose accumulated by it.
Finally, we can "catch" not the secondary effects caused by ionization, but the ions themselves - just like in the ionization chamber, only this chamber is filled not with gas, but with a semiconductor - germanium, silicon, cadmium telluride, and finally - diamond. The average current through the detector will be proportional to the power of the dose absorbed by it.
But what about the well-known Geiger counter? But he does not measure the dose. He can only react with impulse to the passage of particles through it, not understanding what flew into it, nor what kind of energy it had. That is, he can measure such a characteristic of particle flux as fluence : how many particles flew through a given area. A scintillation or semiconductor detector will work in exactly the same way if we only record the fact of the appearance of a pulse, ignoring its amplitude.
Dose in different materials and stroke with stiffness
In the paragraph on the absorbed dose, I mentioned in passing that the dose absorbed by different materials in the same radiation flux will be different and will depend on the energy of quanta and the properties of the substance. In the case of gamma radiation, its absorption is determined by the only characteristic of the material - the average (or effective) atomic number

The figure (from the New Handbook of a Chemist and Technologist, vol. 11, p. 111) shows the energy dependences of the dose sensitivity of detectors made on the basis of different scintillators. Anthracene (lighter in average atomic weight than living tissue) and sodium iodide (significantly more “heavy” than the last) are compared on the left. It can be seen that in a certain energy range, the sodium iodide-based detector overestimates the dose by 10 times! And on the right graph it is shown that taking a mixture of organic scintillators - more “light” and more “heavy” than living tissue, you can almost completely eliminate the “move with stiffness”.
Another way to eliminate the “stroke with stiffness” is to select filters that absorb radiation in the area where the sensitivity of the detector is excessive.
Conclusion
In conclusion, I will give a small plate, which summarizes the main quantities considered in the article.

And for a more complete familiarization with the topic, I recommend the lectures of Professor Igor Nikolaevich Bekman , Moscow State University
All articles in the series
Radiation: Everyday life of the radiochemical laboratory
Radiation: sources
Radiation: risks, safety, protection